Using data collected by NASA’s Kepler Space Telescope, scientists have found four free-floating, or rogue, planets near the core of the Milky Way. These planets formed in discs in other planetary systems and were thrown out by gravitational interactions with larger planets. Plus, the early solar system, including ancient Earth, Jupiter’s chemistry, and Mercury’s core.
Podcast
Transcript
Hello and welcome to the Daily Space. I am your host Dr. Pamela Gay.
And I am your host Beth Johnson.
And we are here to put science in your brain.
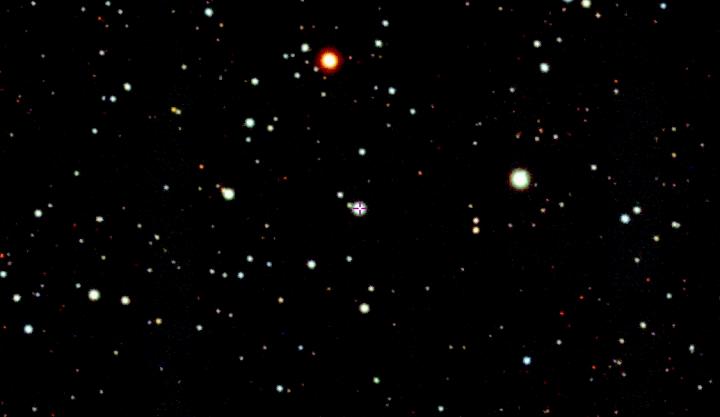
Astronomy is in many ways the greatest “whodunnit” mystery of all. Consider the star SMSS J200322.54-114203.3. This elder star is polluted with heavy elements in amounts that can’t be explained by any known event. These elements – zinc, uranium, europium, and possibly gold – have ratios that don’t match what is produced in normal supernovae or even during the collisions of neutron stars. And their amounts are so low, that they likely all came from a single event that polluted the batch of primordial gas that formed this star.
According to lead author David Yong: The star we’re looking at has an iron-to-hydrogen ratio about 3000 times lower than the Sun – which means it is a very rare: what we call an extremely metal-poor star. However, the fact that it contains much larger than expected amounts of some heavier elements means that it is even rarer – a real needle in a haystack.
But since the star exists, something had to have produced these elements. To quote Sherlock Holmes: When you have eliminated the impossible, whatever remains, however improbable, must be the truth.
There is one additional kind of element-forming star death: the explosion – a hypernova – of an early generation star that was extremely massive and consisted of pretty much only those elements formed in the Big Bang. In a new paper published in Nature, Yong and his team describe how an early massive star, given enough spin and a large enough magnetic field, would be able to produce all the elements seen.
This star, SMSS J200322.54-114203.3, is helping us learn new things about those early stars. Those factors – had to be spinning and had to have a magnetic field – are new constraints on objects we’ve never seen. Astronomers may not use magnifying glasses, but armed with sufficiently powerful telescopes, we can sleuth out amazing mysteries.
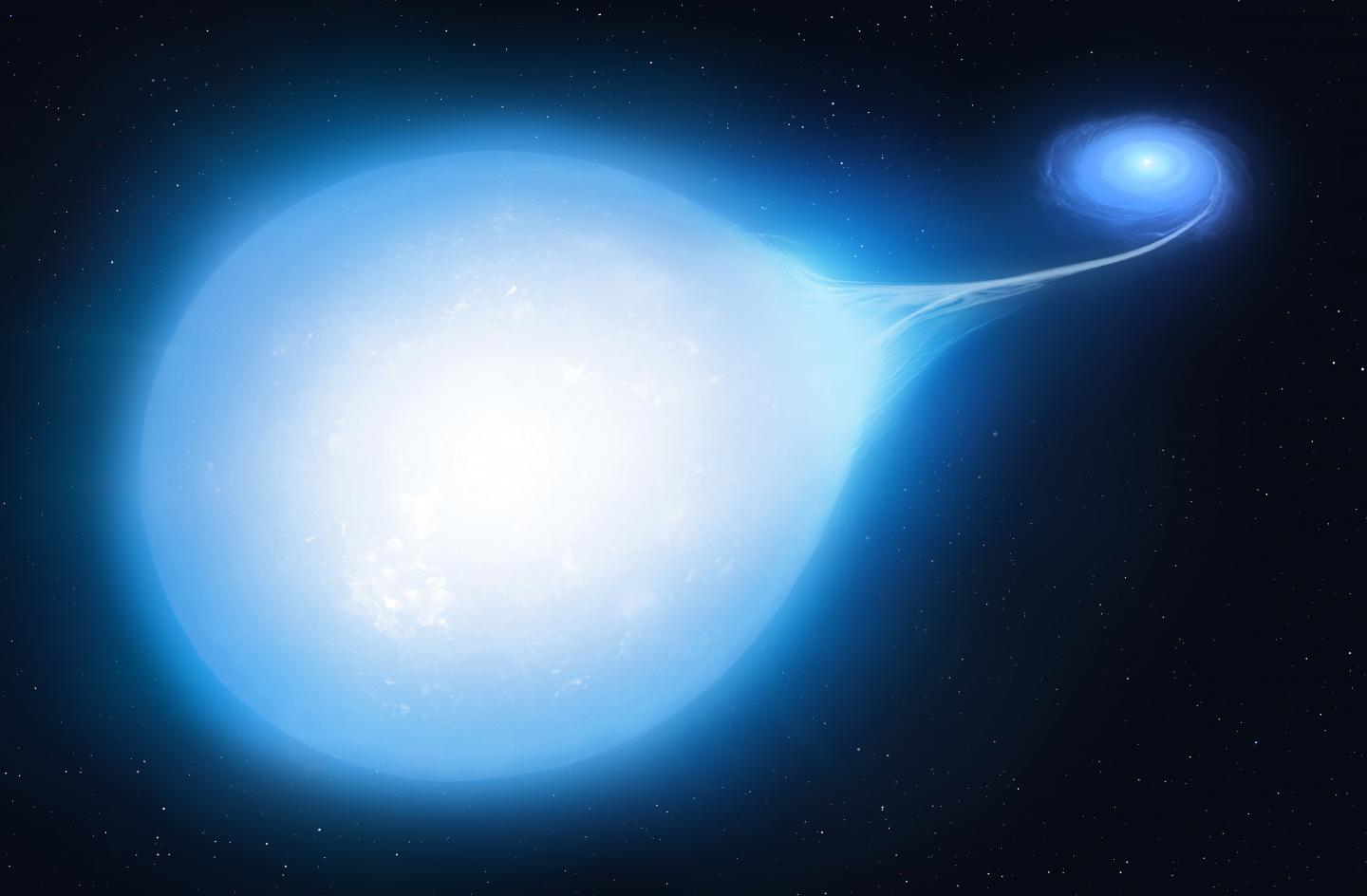
And sometimes, armed with those powerful telescopes, we find things we knew should exist and, it turns out, do exist. Case in point: take the star system HD265435. We can see a hot, tiny subdwarf star, and when we examine it closely, we can see that star moving and changing in brightness in the exact manner you’d expect if a much more tiny white dwarf star were nearby pulling on the subdwarf’s surface.
In a new paper in Nature Astronomy led by Ingred Pelisoli, researchers describe how the subdwarf star’s brightness appears to vary over time ever so slightly and in exactly the way you’d expect if the star was teardrop-shaped instead of round. When that teardrop is edge on to us, we see more of the star and receive more light. When we are instead looking at the rounded top of the tear, we see a smaller area and less light. This distorted star is having its mass gravitationally stolen by the white dwarf, and over time, this is going to allow the white dwarf to grow until one day, roughly seventy million years in the future, the white dwarf has consumed so much matter that it explodes as a Type 1a supernova.
While I don’t think any of us want to wait around to see that future explosion, it’s still exciting to get to watch the early stages of this mass transfer.
At a certain level, the lives and deaths of stars shape everything else visible in our universe, and that everything else likes to shape the patterns of the stars.
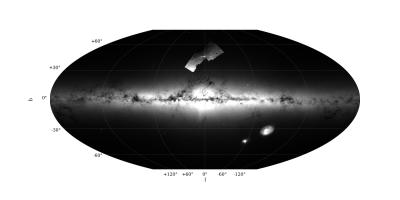
Stars generally form through the collapse of massive clouds of cold gas, and those stars end up forming a cluster of stars. Depending on the location of that cluster, it will get torn apart by gravity in any of a number of different ways, as some stars are pulled more or less than others. Throughout our galaxy, astronomers have found streams of stars that appear to have formed together, either as a single cluster or as a more complicated dwarf galaxy, and as that original object passes through our galaxy, it is stretched apart into a streak.
The thing about this story is it’s just that: a story told by theorists that nicely matches the data. The problem is, according to Mark Gieles: …none of the recently discovered streams have a star cluster associated with them, hence we can not be sure.
To sort if theory matches reality, a stream with an associated cluster was needed. Enter Palomar 5, a globular cluster discovered in 1950 that is extended out into the starts of a stellar stream. This system has an overabundance of black holes and not a lot of stars. Using computer models, a team led by Gieles was able to calculate that the disruption we see Palomar 5 experiencing would preferentially remove normal stars and transform the cluster from a normal distribution of stars and dense objects like black holes to the black hole dominated system we see today. To theorists: you got this!
The universe is filled with weird stuff, in every possible combination. Take planets. We are used to talking about them orbiting stars, and we are used to finding them by looking at how they interact with their star’s light. Planets don’t need stars, however, and during its extended K2 mission, Kepler peered at a field of stars toward the center of the galaxy. This dense region was picked as a high probability location for seeing rogue planets passing in front of background stars.
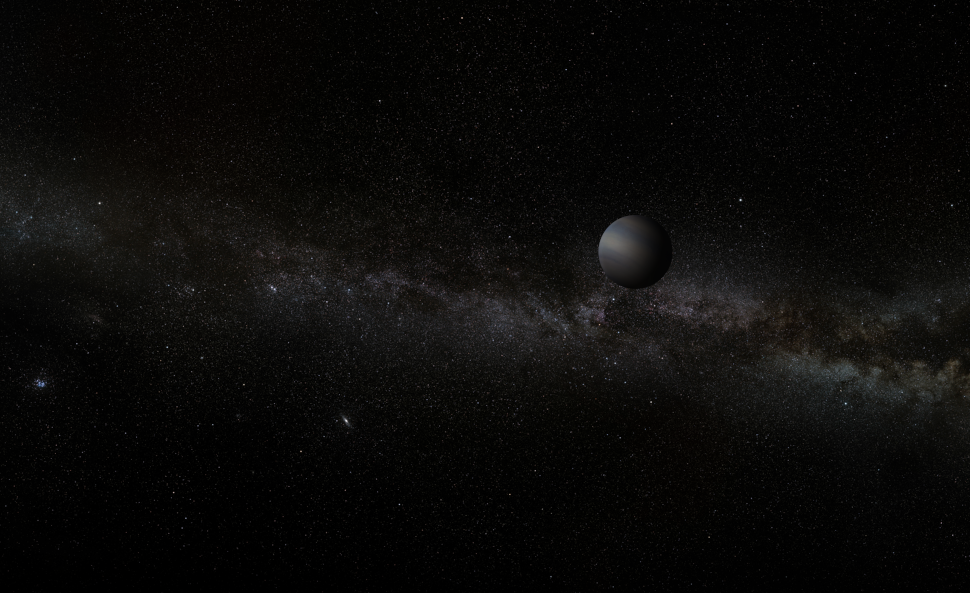
When two objects line up, the gravity from the front object can bend background light from the rear object – light that was meant for some other part of the universe and that we now get to see. This effect is minimal when one human lines up with another human, but it becomes noticeable when large enough planets align with stars. Planets have been found this way from Earth many times, and now we know Kepler could find planets this way, too. In a new paper in Monthly Notices of the Royal Astronomical Society (MNRAS) and led by Ian McDonald, four new free-floating planets have been found in this one field, and 22 planets were co-identified by Kepler and Earth-based surveys.
Let’s put this in perspective. Kepler looked at a patch of sky that contained many, many stars and an unknown number of rogue planets. All those stars and all those planets are ever so slowly moving through the sky as they orbit the galaxy. In 26 cases, the orbits lined up just right for the planet to magnify the amount of light we get. Since we know how many stars we’re looking at and we know how long Kepler looked, it starts to be possible to figure out how common this kind of alignment is. And the answer is that they appear to be very common. The research team teases that they’ll have more precise estimates in their next paper, but the question at this point is becoming “are there more planets orbiting stars or cast out among the stars?”
This raises all sorts of interesting questions about how many worlds solar systems start with, how many collide and merge, and how many simply get thrown away like so much unwanted rock or ice; the Universe isn’t picky. These worlds are destined to be cold, dark, and generally invisible except through their periodic gravitational interactions. While scientifically we can’t observe these worlds in a satisfying amount of detail, knowing they’re out there is food for thought as I dream of having time to write fiction. Here is to hoping someone dreams up a cool future for these lonesome worlds.
There is one planet we can observe in detail: our own Earth. And sometimes we are lucky enough to find rocks from elsewhere here that we can analyze and use to learn about our solar system’s history. Understanding that history can help us understand not only our own solar system but exoplanetary systems as well. Every piece of information we collect can lead to a better picture of solar system formation, something we seem to talk about a lot on Daily Space.
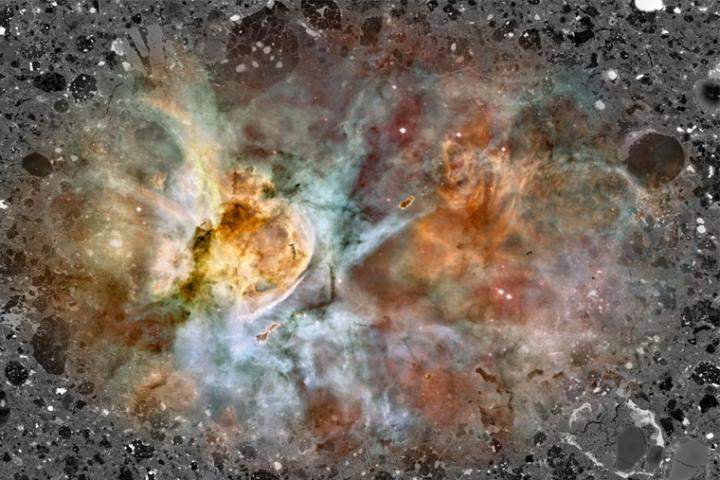
Results published in Geochimica et Cosmochimica Acta show that a small meteorite named Acfer 094, which was found in 1990, is an incredibly primitive meteorite from our early solar system, 4.6 billion years old. By analyzing the sulfur and oxygen isotopes in this 85-gram rock, scientists determined that differences in the chemical composition from our Sun were caused by ancient starlight.
The Sun likely formed where massive stars were relatively close, and ultraviolet light from those massive stars broke apart carbon monoxide gas in our protoplanetary disk and irradiated hydrogen sulfide gas, changing the overall composition from what our star contained. So the building blocks for our solar system were affected by starlight from a nearby massive star.
And we can see evidence of this same effect in proplyds, or protoplanetary disks, found in places like the Orion Nebula, where massive O and B type stars are tearing apart the gas in the disk. That’s a pretty impressive link in the planetary formation chain, and it’s all because of a tiny rock falling to Earth.
Of course, tiny rocks were not the only ones that fell from the sky and impacted the surface of Earth.
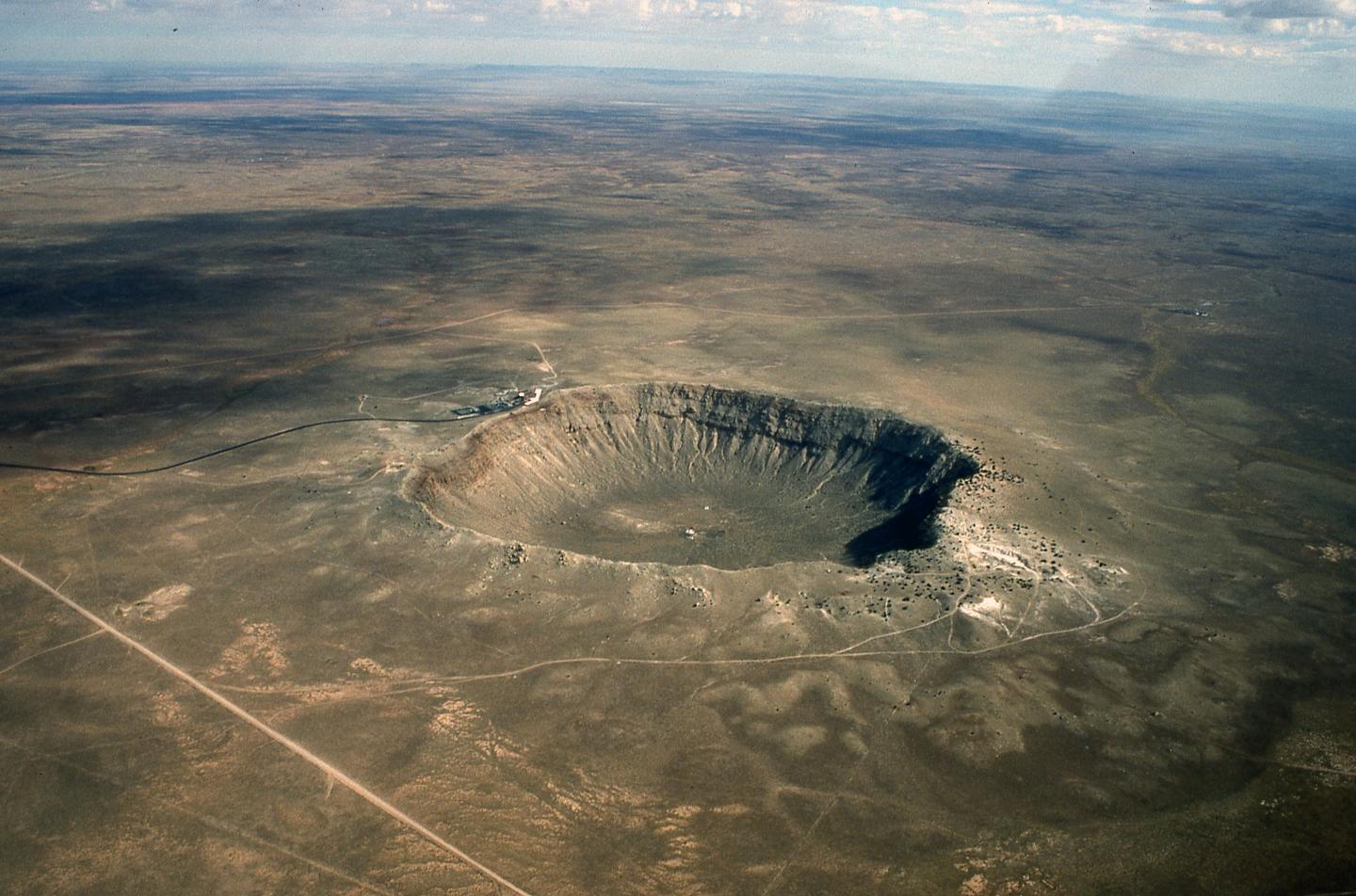
In new research presented at the Goldschmidt Conference, scientists have found that our precious planet was likely hit by ten times more massive impacts in the distant past than previously thought. These impacts would be similar in scale to the asteroid impact that wiped out the dinosaurs 65 million years ago, and they seem to have happened every 15 million years, particularly during a period stretching from 2.5 to 3.5 billion years ago.
The asteroids involved in these impacts would have been greater than ten kilometers in diameter, and their effect on the early Earth would have been different than what happened with those long-gone dinosaurs. The impacts threw molten particles up into the atmosphere and released vapors from under the surface of the Earth, all of which cooled, solidified, and fell back down to be embedded in the rock record. These tiny particles can now be found as glassy spheres in rough layers around the globe at various intervals.
Again, tiny bits of rock tell us more about the history of our solar system, and how amazing is that? Especially because, unlike drier bodies such as the Moon and Mars, the evidence of these impacts go through weathering and plate tectonics, making them incredibly difficult to find.
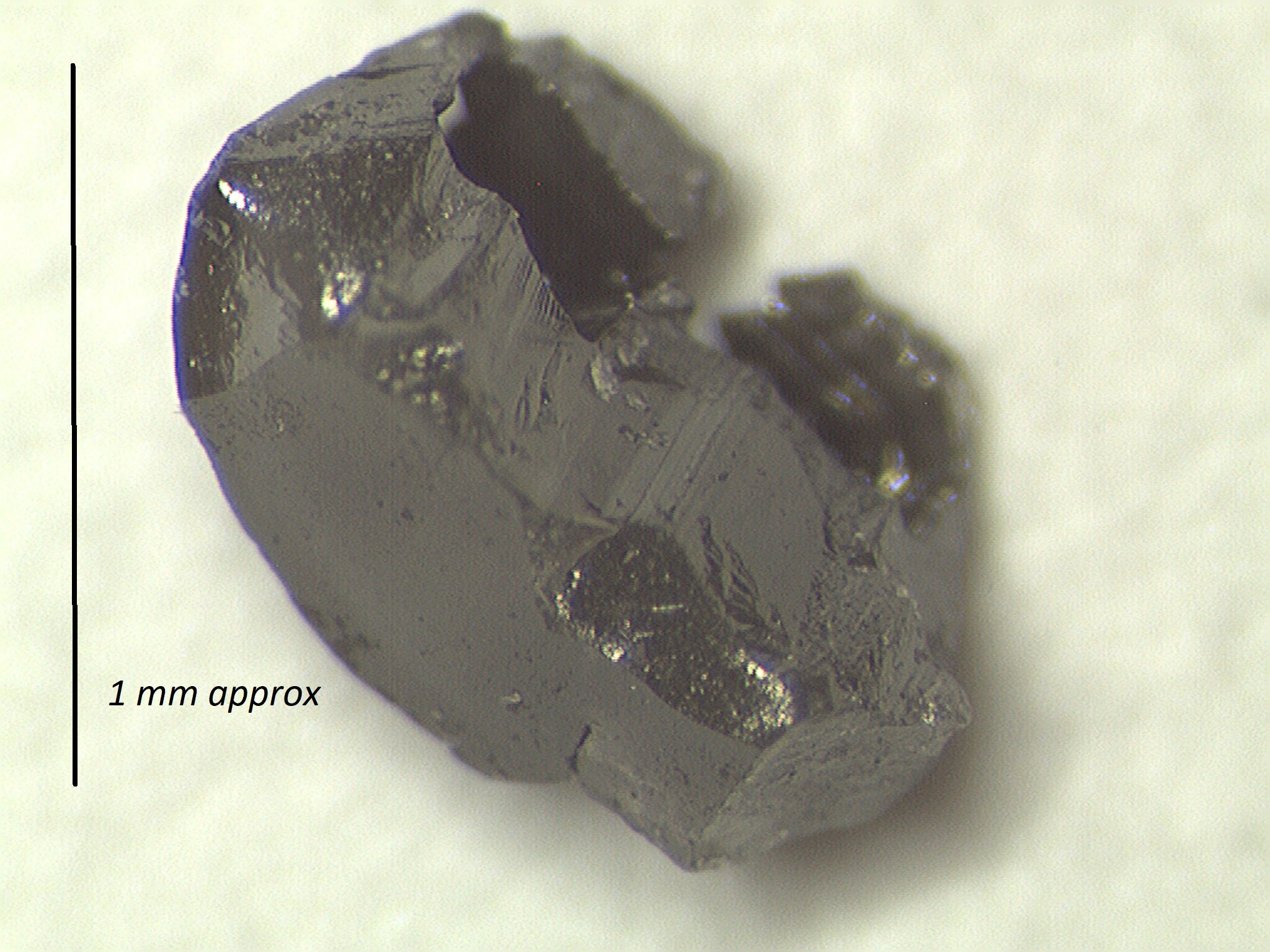
But wait, there’s more! In another presentation at the Goldschmidt Conference, researchers explained how ancient diamonds were used to determine the composition of Earth’s atmosphere. They found that at least 2.7 billion years ago, our planet was primed and ready for an explosion of life. The volatile gases trapped in those diamonds, such as hydrogen, nitrogen, neon, and various carbon molecules, were found to exist in similar proportions to what we find in our current mantle. Basically, the conditions necessary for life were here billions of years ago and haven’t fundamentally altered since. Diamonds, it turns out, make amazing time capsules for us to use in studying our planet’s distant past. One of the benefits of being the hardest mineral on the Mohs scale is that they’re almost indestructible. So maybe diamonds are actually a planetary scientist’s best friend…
One of the first rules of astronomy is humility. We don’t phrase it that way. Instead, we say there is a cosmological principle, and it says we don’t live in either a special time or a special place, and if you look at a large enough chunk of the universe, everything really is the same everywhere. The thing is that sometimes we take this rule too far, and we assume even our planets are pretty normal.
Folks, I’m starting to believe there is no such thing as normal.
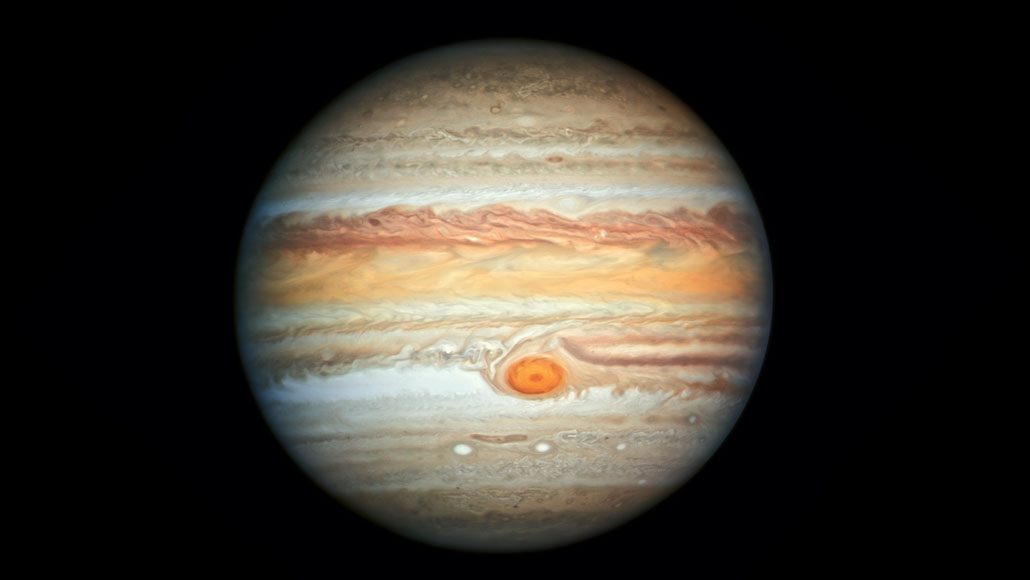
Jupiter. We constantly compare other worlds to Jupiter like it was a normal measure of a planet. There are hot Jupiters, super Jupiters, sub-Jupiters, cold Jupiters. There is a laundry list of adjectives used to describe worlds as they relate to Jupiter as if it were the normal one. It is not normal, not even in our own solar system.
Jupiter is the most dramatically colorful world in our solar system, and these colors come from its rich mixture of elements – elements that include xenon, krypton, argon, and nitrogen. In general, these elements weren’t all that common where Jupiter is today when the planets were forming, and the closer one got to the Sun, the fewer of these gases were around. This strange reality – that Jupiter has these elements, and yet, these elements like it better in the outer solar system – led two different teams in 2019 to speculate that Jupiter may have formed in the outer solar system and spiraled in.
Except we had already built this cool model of Jupiter and Saturn starting closer in and moving out, and do we really want to play yo-yo with planets? Don’t answer that. I know I actually would totally play yo-yo with Jupiter if I could.
Anyway, not wanting to fling Jupiter around more than necessary, another research team has come up with a new idea: what if a pile-up of dust and debris cast a shadow that let Jupiter form where it was both cool and close?
Midway through the asteroid belt was a snow line. Inside that line, water was liquid, and at that line, just like in a winter storm, the phase changed and things got messy. Imagine if, at this line, a broad ring could form that cast a shadow big enough to allow Jupiter to lurk in the shadow, grabbing up weird elements, and preparing for its colorful future. It’s a cool picture, and while we may never know if it’s real, it’s kind of amazing to know that the science is possible.
This work appeared in Astronomy and Astrophysics and was performed by Kazumasa Ohno and Takahiro Ueda.
Before we go, we have one last tale and a press release that seems to be asking Mercury some rather personal questions.
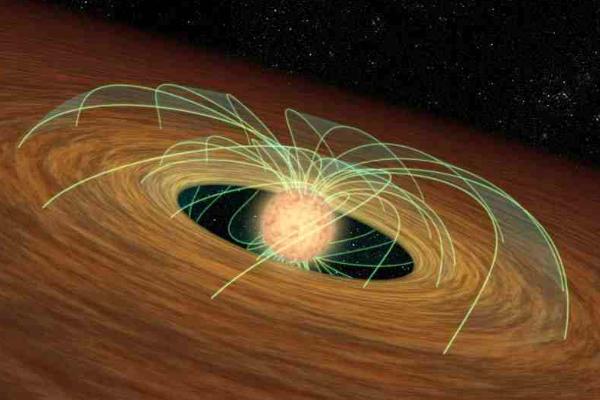
In new research shared out by Tohoku University, researchers ask, “Why does Mercury have a big iron core?” It turns out that this tiny planet has a metallic core making up about three-quarters of its mass. If Earth were built the same way, gravity would be much stronger and in general, we’d enjoy our world a lot less. In trying to understand how our world and Mercury ended up so different, researchers looked at both the building blocks of planets and the conditions in the early solar system.
Planets are largely a mix of the same stuff that makes up meteorites: iron, silicon, carbon, oxygen, and magnesium all mixed together. In the early solar system, the Sun had a strong magnetic field that interacted with all the grains and small objects in the protoplanetary disk, and the iron in that disk did what iron does in a magnetic field: it migrated toward the magnet. This made the volume near the Sun where Mercury formed extra iron-rich, allowing Mercury to be all that much denser than Earth.
The moral of the story: if you want to shine in all the colors of the rainbow, form in shadow, but if you want to be a dense lump, the spotlight might be the place to be, at least for planets. Your results may vary.
This has been the Daily Space.
Want more of us? Check out CosmoQuest.org and get tickets for CosmoQuest-a-Con, our 80s space party that will take place July 16-18.
Learn More
New Hypernova Type Explains Stellar Mystery
- ASTRO 3D press release
- “r-Process elements from magnetorotational hypernovae,” D. Yong et al., 2021 July 7, Nature
Impending Supernova Located by Teardrop Star
- University of Warwick press release
- W.M. Keck Observatory press release
- “A hot subdwarf–white dwarf super-Chandrasekhar candidate supernova Ia progenitor,” Ingrid Pelisoli et al., 2021 July 12, Nature Astronomy
Star Cluster Found to Have Huge Population of Black Holes
- University of Barcelona press release
- “A supra-massive population of stellar-mass black holes in the globular cluster Palomar 5,” Mark Gieles et al., 2021 July 5, Nature Astronomy
Rogue Planets Found Near Milky Way’s Core
- RAS press release
- The University of Manchester press release
- Astronomers Spot a Batch of Rogue Planets Near the Galactic Core (Gizmodo)
- “Kepler K2 Campaign 9 – I. Candidate short-duration events from the first space-based survey for planetary microlensing,” I McDonald et al., 2021 July 6, Monthly Notices of the Royal Astronomical Society
Primitive Meteorite Hints at Solar System’s Past
- Washington University in St. Louis press release
- “Cosmic symplectite recorded irradiation by nearby massive stars in the solar system’s parent molecular cloud,” Lionel G. Vacher et al., 2021 June 25, Geochimica et Cosmochimica Acta
Massive Impacts Happened 10x More Often Than Thought
Ancient Diamonds Contain Volatiles Similar to Today’s Mantle
- Ancient diamonds show Earth was primed for life’s explosion at least 2.7 billion years ago (EurekAlert)
Jupiter formed in the shadow of snow
- A shadowy birthplace may explain Jupiter’s strange chemistry (Science News)
- “Jupiter’s “cold” formation in the protosolar disk shadow,” Kazumasa Ohno and Takahiro Ueda, 2021 July 1, Astronomy & Astrophysics
Mercury’s Metal Core is Yuge
- Tohoku University press release
- University of Maryland press release
- “Terrestrial planet compositions controlled by accretion disk magnetic field,” William F. McDonough and Takashi Yoshizaki, 2021 July 2, Progress in Earth and Planetary Science
Credits
Written by Pamela Gay and Beth Johnson
Hosted by Pamela Gay and Beth Johnson
Audio and Video Editing by Ally Pelphrey
Content Editing by Beth Johnson
Intro and Outro music by Kevin MacLeod, https://incompetech.com/music/