Researchers found that among about 50 supernovae, many had nearly identical spectra, paving the way for making more accurate distance calculations. These calculations, in turn, open up the possibility of using supernovae to better search for dark energy. Plus, OSIRIS-REx, Voyager I, planetary formation, and volcanoes on Mars.
Media
Transcript
Hello and welcome to the Daily Space. I am your host Dr. Pamela Gay.
And I am your host Beth Johnson.
And we are here to put science in your brain.
Here on Earth, we often say form follows function. A long-necked giraffe can eat leaves from treetops. The airfoil edge of a racing yacht can cut through the air while generating speed. We can look at forms and see how the shape is designed for action or at least artistry.
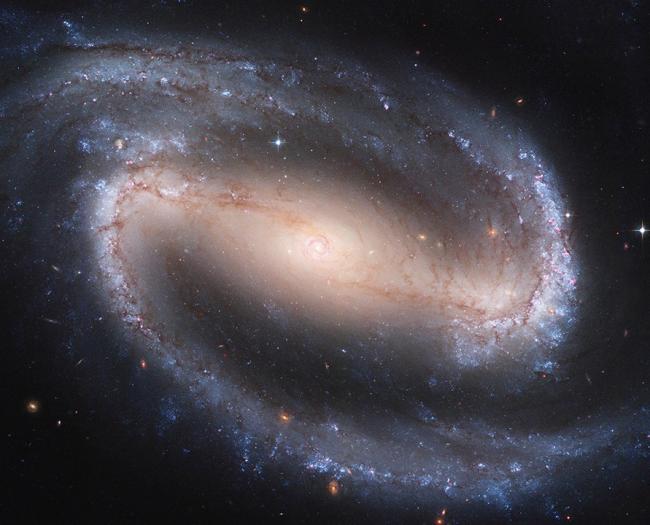
When we look out at the universe, we are confronted by shapes that aren’t generated through the interactive design of evolution or engineering. When we see a spiral galaxy with a strong bar-like structure in the center, we don’t see something whose shape is designed with purpose; rather we see something shaped by gravity and light whose shape may have consequences, and as astronomers, when we see these shapes we make it our job to figure out both how they formed and what they do.
We’ve known for a long time that bars are associated with a spiral galaxy having a companion galaxy. The exact details of how that companion makes a gorgeous bar of stars is a matter for computer simulations. Understanding what is going on is complicated by the obscuring dust and glowing gas that makes it impossible to peer into a galaxy using the colors of light our eyes see.
In a new study appearing in Astronomy & Astrophysics, researchers led by Eduardo González-Alfonso have switched to using infrared light. What we’re learning now is that a bar can act as a highway to funnel gas and dust from the outer parts of the galaxy into the core, where it can trigger star formation and feed the central supermassive black hole. In this new study of the barred spiral NGC1300, astronomers used infrared telescopes to look through that dust and gas to map the densities and temperatures of material within the galaxy. Specifically, they looked at this galaxy’s water vapor and the specific colors of light it gives off. Since movement actually changes the color of light a bit, with things appearing redder as they move away and bluer as they approach, they could map the motions of the water vapor using these color shifts.
Their work focused on the inner 450 light-years of the galaxy and showed the galaxy has three components: a warm envelope, a nuclear disk, and a tiny, much warmer compact core. This region is starbursting at a rate of eighteen solar masses of new stars a year, while material is also streaming into the system’s black hole. It is unclear how much of this is a snapshot of the galaxy at this moment and how much is a long-term aspect of this structure. Our galaxy is weakly barred and just not this interesting, but it might have been in the past and could be again in the future.
At the end of the day, all we can do is take snapshots of the universe as we see it and use those snapshots to try and puzzle out the past and future of our universe and the stuff inside. One little spacecraft didn’t realize just how hard this would be when it lifted off for the near-earth asteroid Bennu in 2016.
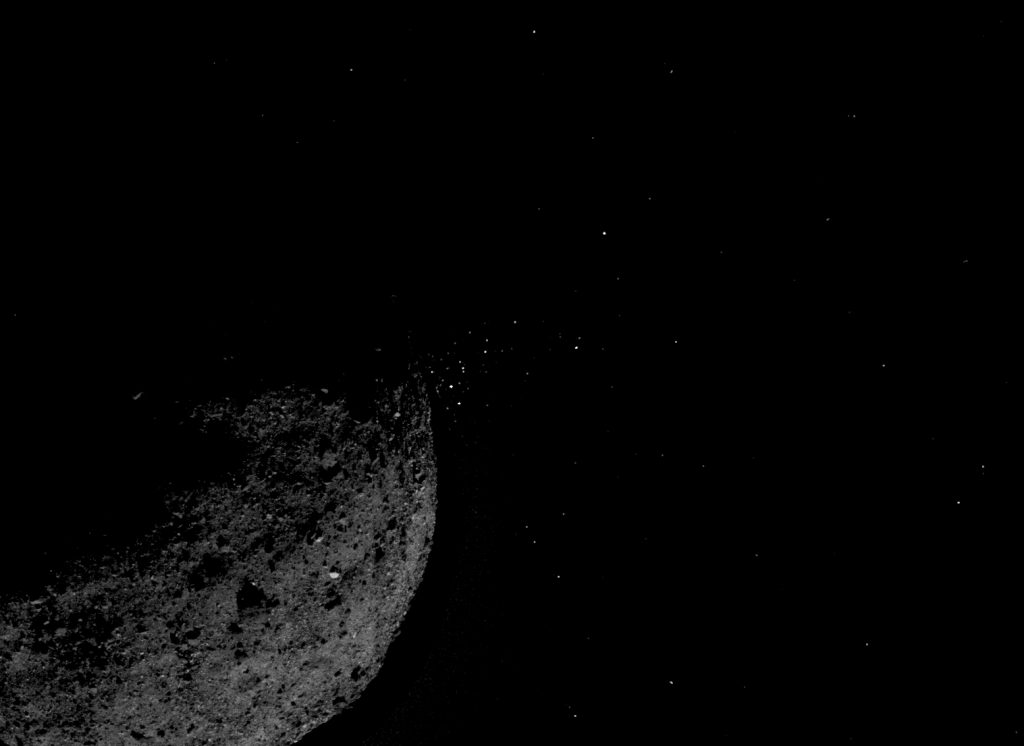
The OSIRIS-REx mission has captured both images and a sample of Bennu, and this data tells the story of a violent past of collisions with rock-shattering forces. While the mission could beam those images to Earth as radio signals, the rocks, those have to be carried back, and we are pleased to share that on May 10, OSIRIS-REx started that long journey home and is slated to fling its rocks our way for a September 24, 2023, arrival in Utah.
It’s unknown what the mission might be called upon to do in the future, but the current plan is for OSIRIS-REx to fly past Earth, fling its rock samples our way, and then continue into an orbit around the Sun inside of Venus’ orbit. According to Mike Moreau, the OSIRIS-REx deputy program officer: There’s a lot of emotion within the team about departure. I think everyone has a great sense of accomplishment because we faced all these daunting tasks and were able to accomplish all the objectives thrown at us. But there’s also some nostalgia and disappointment that this part of the mission is coming to an end.
For the past three years, OSIRIS-REx has proven over and over that it can do more than anyone ever expected they’d have to ask of it. Bennu turned out to be a mess of an asteroid that is throwing bits of rock away from its surface on a regular basis. With no particularly safe place to take a sample, and the active tossing of rocks at the spacecraft, the mission team had to sort “is Bennu dangerous to orbit” and then jump to solving “how do we land with no clear areas?”
Luckily, it was safe to orbit, and the team was able to program new self-driving routines into the spacecraft so it could drive itself to a safe-enough sample site. It has been an impressive few years, and now the long wait for the rocks begins.
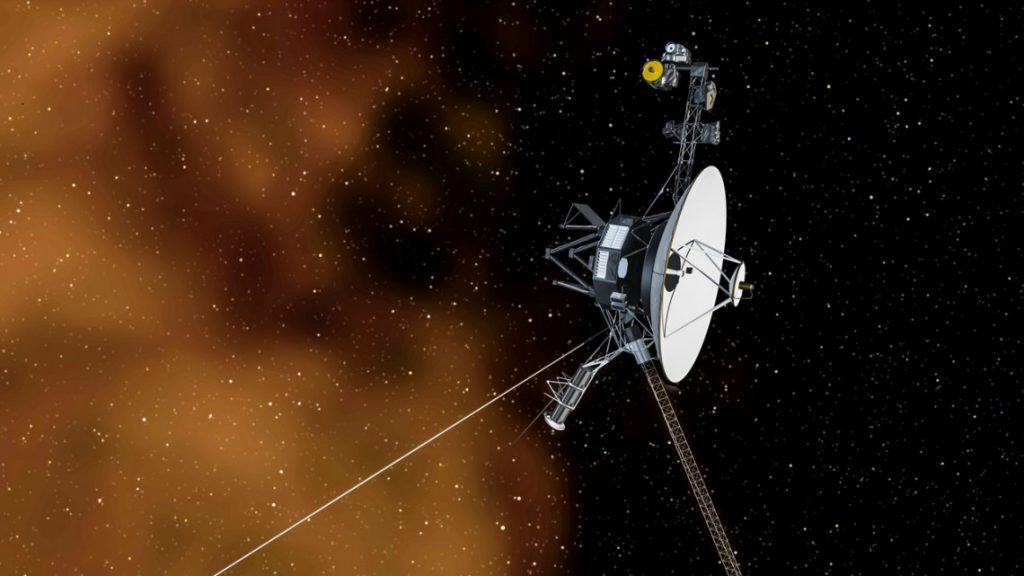
Space is not silent. At least not if you know how to listen. The Voyager I spacecraft has now departed our solar system, and as it flies through interstellar space, it’s detecting the steady drone of plasma waves. Like the hum of fluorescent bulbs, this hum comes from excited atoms. In this case, the gas is super low density and extends at least 10 AU. This hum is in addition to the loud bursts associated with solar activity exciting the gas and seems to be caused by thermal processes or quasi-thermal noise. These new results are published in Nature Astronomy by a team led by Stella Koch and show that even middle-aged spacecraft have good science they can do.
It’s not often that we get to talk about experimental research into exoplanets and astrobiology. In new research published in Nature Geoscience, researchers used the high-pressure lab at Rice University to understand how nitrogen is attracted to metallic planetary cores. Lead author Damanveer Grewal explains: We simulated high pressure-temperature conditions by subjecting a mixture of nitrogen-bearing metal and silicate powders to nearly 30,000 times the atmospheric pressure and heating them beyond their melting points. Small metallic blobs embedded in the silicate glasses of the recovered samples were the respective analogs of protoplanetary cores and mantles.
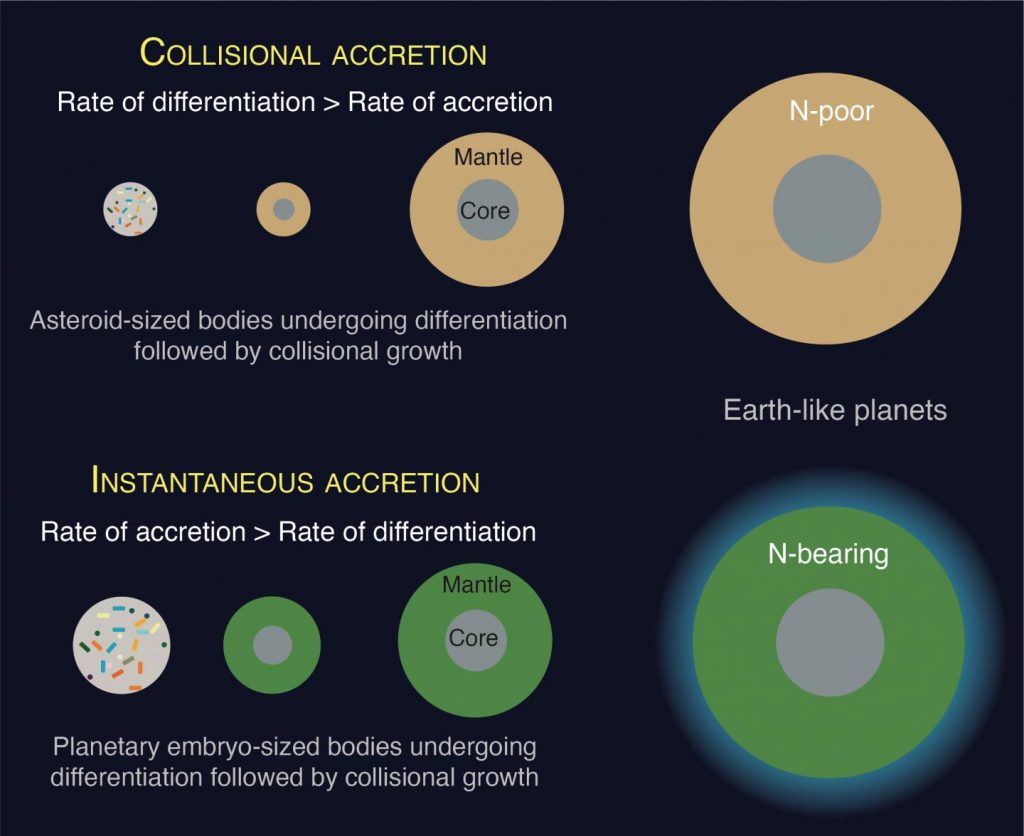
By analyzing the data, the team was able to model how nitrogen gets distributed between the atmosphere, mantle, and core. Grewal continues: We realized that fractionation of nitrogen between all these reservoirs is very sensitive to the size of the body. Using this idea, we could calculate how nitrogen would have separated between different reservoirs of protoplanetary bodies through time to finally build a habitable planet like Earth.
Basically, to get the amount of nitrogen we have here on Earth, which life here needs to survive, your planet needs to grow more quickly than it differentiates out into crust, mantle, and core. Following that path leads to the accretion of more nitrogen, which apparently loves the metallic liquid that forms the cores of rocky planets. This work, of course, can be applied to exoplanets as well, if we can figure out their composition.
Nitrogen isn’t the only material we’re looking for when studying planetary formation. An international research team has discovered methanol in a protoplanetary disk. The gas was found in the warmer part of the disk, which means it cannot have formed there and must have come from the surrounding cold gas clouds that gave birth to the star and the disk. This work was published in Nature Astronomy and shows that methanol has to be inherited and not created; a process that, if common, may give life in other systems a head start.
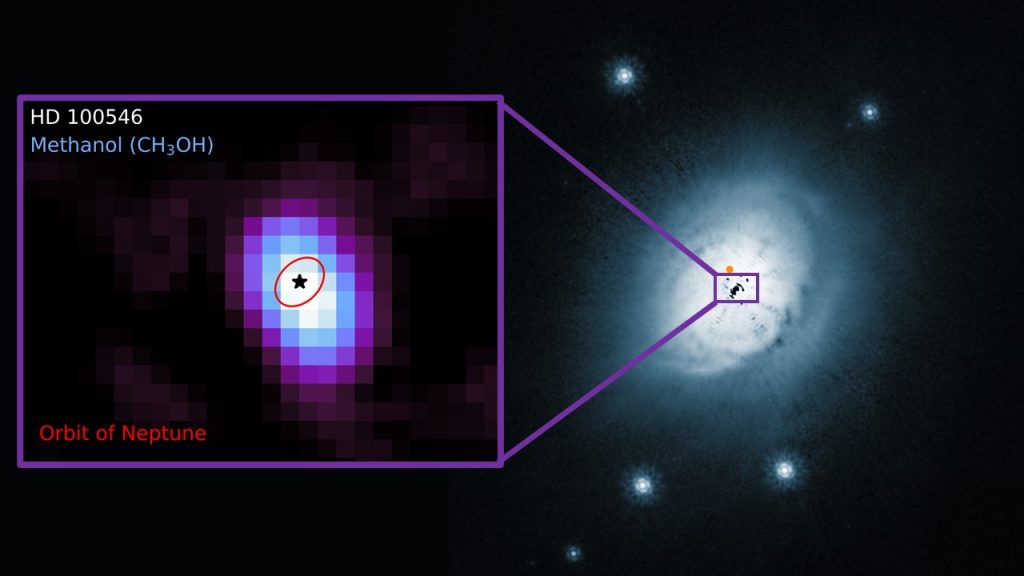
Per the press release: Methanol, CH3OH, is one of the simplest complex molecules. It is considered by astronomers to be a precursor for the pre-biotic chemistry essential for life because it can be used to form, for example, amino acids and proteins.
And lead author Alice Booth explains: This is a very exciting and surprising result. Whilst warm methanol has been detected in the warm, young disks, because of the nature of this disk this is the first clear observational evidence that complex organic molecules can be ‘inherited’ from the earlier cold dark clouds phase.
The team’s next goal is to gather more data and possibly find even more complex oxygen-bearing molecules in their search for more pre-biotic chemistry. We’ll keep you updated here on the Daily Space as those results are reported.
In the meantime, while some scientists are trying to understand just how life could come to be and what ingredients are necessary to make that happen, others are continuing in the quest to understand the hows of planetary formation. A new paper in The Astrophysical Journal examined the formation of rings in protoplanetary disks and found that planets may begin the process of accreting dust and gas far earlier in a star system’s life than previously thought.
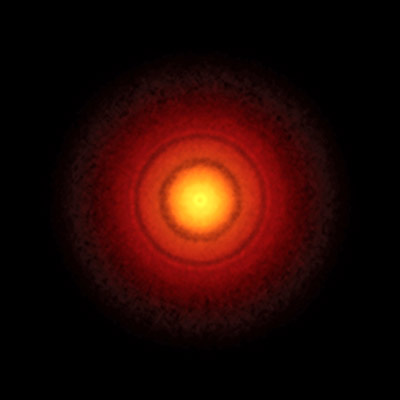
Rather than using experimental observations, this team used computer simulations to model various planetary formation scenarios. Their results indicate that the dust may begin to come together into larger particles during the star’s protostellar stage. This means that the planets in the system are forming before the star is done forming. Lead author Satoshi Ohashi notes: It’s really surprising because during planet formation the dust grains should stay in the disk, but material is still falling into the central star during the protostellar stage. So we are thinking that planet formation could be a highly dynamic process.
The team compared their simulation results to the observations of 23 different ring structures observed using the Atacama Large Millimeter/submillimeter Array (ALMA) in Chile and found the two data sets agreed. We will get this planetary formation process figured out, y’all. Some day.
From star formation to planet formation, we now turn to the end of a massive star’s life and look at supernovae.
It’s long been realized that one kind of supernova – the explosion of white dwarfs that have too much mass dumped on them – should have consistent explosions, in much the same way that blowing up to identical sticks of dynamite should create identical explosions. As with so many things, that word “should” is the kicker. If the supernovae do all have the exact same energy, we can use them to measure distance by looking at the relationship between known luminosity and measured brightness. By then measuring the motions of these supernovae, we can get at the expansion rate of the universe.
It was from supernovae that in 1998 folks discovered our universe is accelerating, and that is an uncomfortable realization. If it turns out that the supernovae simply change over time, with distant supernovae being a slightly different luminosity, then our data will artificially look like the universe is doing something it is not.
Since 1998, a whole lot of effort has gone into trying to understand how local and distant supernovae may or may not differ from one another. In two new papers in The Astrophysical Journal, both led by Kyle Boone, researchers use data from the Nearby Supernova Factory, a survey of supernovae explosions. With this data, they can make detailed comparisons of the supernova spectra, as a function of time. According to Boone: Conventional measurement of supernova distances uses light curves – images taken in several colors as a supernova brightens and fades. Instead, we used a spectrum of each supernova. These are so much more detailed, and with machine-learning techniques it then became possible to discern the complex behavior that was key to measuring more accurate distances.”
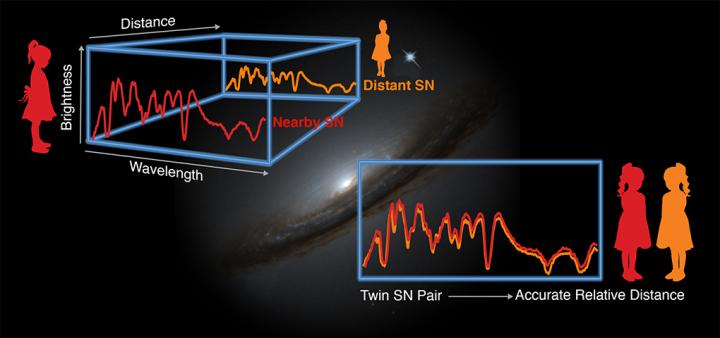
In this immense data set, pairs of identical-looking supernovae at near and far distances could be found. They also found that once they’d corrected their data for brightness and color, variability in supernovae was restricted to specific spectral lines, and by taking into consideration those specific differences, they could explain almost 90% of the observed variation in supernovae.
This work gave them two different pathways to test our understanding of the expansion of the universe. Since they had found identical-looking supernovae at very different distances, they could assume the supernovae with identical spectra also had identical luminosities and calculate their relative distances. With enough twins, more and more of space can be compared. They were also able to take into consideration how the observed differences affect the supernovae’s luminosity and make corrections. They estimate their results are accurate to within a remarkably low 3%. According to co-author Greg Aldering: …not only is this distance measurement technique more accurate, it only requires a single spectrum, taken when a supernova is brightest and thus easiest to observe – a game changer!
Our ability to take spectra of supernovae is only limited by the size of the telescopes we are using. It doesn’t require that much light to use filters and measure an object’s brightness in red, blue, and ultraviolet or some other broad set of colors. It is much harder to spread that light out into a detailed rainbow and get enough light in each color that a camera can measure. As larger and larger telescopes are built in the coming years, we will be able to press out further into the universe as we use this new technique to expand our understanding of the universe’s growth.
In our last pair of stories today, I’m going to bring us back closer to home to talk about Mars. Specifically, volcanoes on Mars, so these stories have something for both Pamela and me.
First up, a new study in the Journal of Geophysical Research: Planets highlights the possible importance of small volcanoes on Mars. Most of us are familiar with the gigantic Olympus Mons that dominates the Martian landscape. It’s the tallest volcano in our solar system, reaching up a staggering 23 kilometers. And it’s not alone. There are three other giant volcanoes nearby in the Tharsis Montes. Much of the planetary science work done on volcanic Mars has focused on these titans.
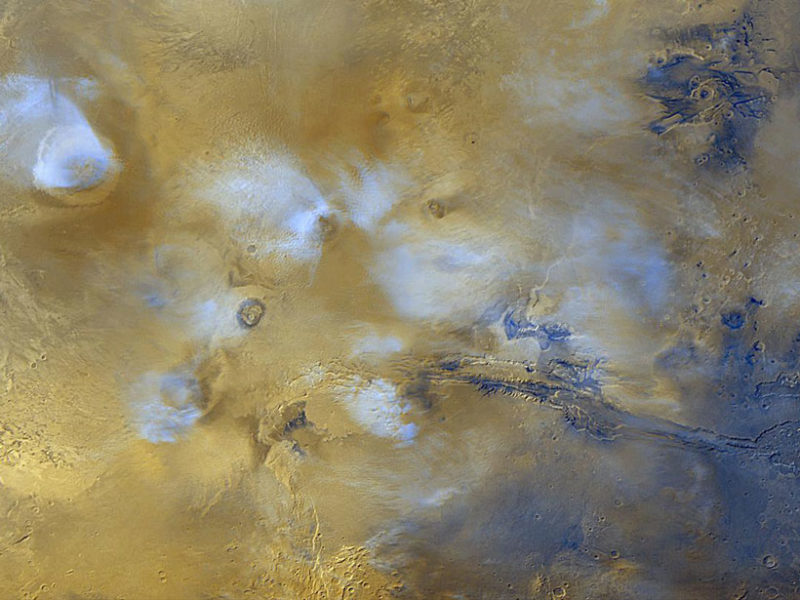
The Tharsis Montes are part of the Tharsis Volcanic Province, and this research team, led by Jacob Richardson, identified over 1,000 small volcanic vents and cinder cones in this area about the size of Africa. These fissures and cones could be analogs to the ones we’ve been watching on YouTube over in Iceland. And they range in age from three billion years to 250 million years, suggesting that a new volcanic vent formed every three million years or so.
Now, Mars doesn’t have plate tectonics as we do here on Earth, and these vents don’t follow Earth formation rules. Here, vents and cones tend to be connected to a larger volcano, forming on its flanks or very close by. If you look at the shield volcanoes of Hawaii or the peaks of the Andes, you can see these vents and cones everywhere. On Mars, however, the vents and cones are not on the flanks of those giant volcanoes; instead, they are scattered to the east and may have had their own source. In fact, they could have contributed as much lava by volume as the bigger volcanoes did!
The difference in formation may have to do with existing fractures in the crust. It appears that the larger volcanoes formed where there were huge fractures, so the magma had clear pathways to the surface. Meanwhile, the volcanic field is not very fractured, so the volcanoes are small. And all of this information helps give us a clearer understanding of Mars’s mantle and possibly even a start on explaining why Earth was the only one of Venus, Mars, and Earth to develop plate tectonics.
Amazingly, while I said these volcanoes date back only as early as 250 million years ago, there has been evidence that volcanism on Mars continued until about three million years ago. And a new paper published in Icarus provides evidence that there was volcanic activity as recently as 50,000 years ago, which is the blink of an eye, geologically speaking.
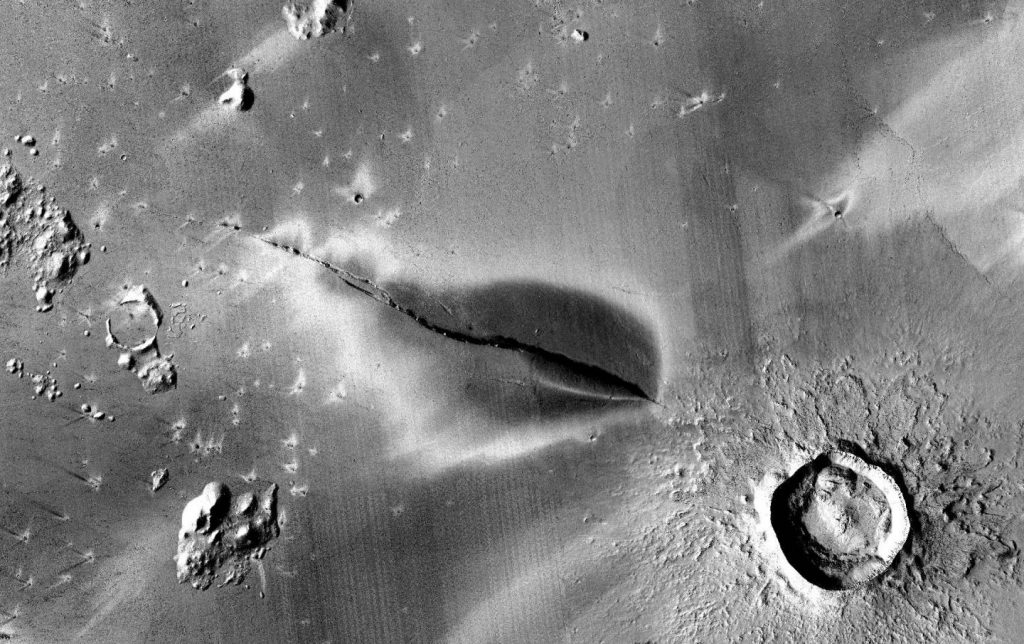
Researchers discovered a previously unknown volcanic deposit in the satellite imagery from various satellites. The eruption produced a fissure that is twenty miles long and eight miles wide, and it’s surrounded by a dark deposit. Further analysis of the properties of the material shows that the eruption likely was similar to a pyroclastic eruption here on Earth: explosive and driven by expanding gases. Think of how a shaken can of soda fountains abruptly when you pop the top.
It’s also possible that the cause of this eruption might be related to the marsquakes being detected by NASA’s Insight lander. We’ve reported before on how the lander’s seismometer detected several large quakes in the Cerberus Fossae region, and that is the same region where this fissure was found. Lead author David Horvath from our own Planetary Science Institute noted: The young age of this deposit absolutely raises the possibility that there could still be volcanic activity on Mars, and it is intriguing that recent Marsquakes detected by the InSight mission are sourced from the Cerberus Fossae.
Magma could still be moving underground, y’all. On Mars. I can’t wait to learn more.
For now, though, this has been the Daily Space.
Learn More
Galactic Bars May Funnel Material and Trigger Star Formation
- Center for Astrophysics press release
- “A proto-pseudobulge in ESO 320-G030 fed by a massive molecular inflow driven by a nuclear bar,” Eduardo Gonzalez-Alfonso et al., 2021 January 7, Astronomy & Astrophysics
OSIRIS-REx Begins Long Journey Home With Rock Samples
- NASA press release
Voyager 1 Hears Plasma ‘Hum’ in Interstellar Space
- Cornell University press release
- “Persistent plasma waves in interstellar space detected by Voyager 1,” Stella Koch Ocker et al., 2021 May 10, Nature Astronomy
Nitrogen Accretion Depends on Style of Planet Formation
- Rice University press release
- “Rates of protoplanetary accretion and differentiation set nitrogen budget of rocky planets,” Damanveer S. Grewal, Rajdeep Dasgupta, Taylor Hough, and Alexandra Farnell, 2021 May 10, Nature Geoscience
Minty Fresh Planetary Disk Contains Menthol
- NOVA press release
- “An inherited complex organic molecule reservoir in a warm planet-hosting disk,” Alice S. Booth et al., 2021 May 10, Nature Astronomy (preprint on arxiv.org)
Planet Formation May Begin As Stellar Formation Continues
- RIKEN press release
- “Ring Formation by Coagulation of Dust Aggregates in the Early Phase of Disk Evolution around a Protostar,” Satoshi Ohashi et al., 2021 February 1, The Astrophysical Journal
Discovery of Twin Supernovae Could Open Up Dark Energy Experiments
- Berkeley Lab press release
- “The Twins Embedding of Type Ia Supernovae. I. The Diversity of Spectra at Maximum Light,” K. Boone et al., 2021 May 6, The Astrophysical Journal
Small Volcanoes on Mars May Have Been A Big Deal
- Eos article
- “Small Volcanic Vents of the Tharsis Volcanic Province, Mars,” J. A. Richardson, J. E. Bleacher, C. B. Connor, and L. S. Glaze, 2021 February 3, JGR Planets
Martian Volcanoes May Still Be Active
- PSI press release
- University of Arizona press release
- “Evidence for geologically recent explosive volcanism in Elysium Planitia, Mars,” David G. Horvath et al., 2021 April 21, Icarus
Credits
Written by Pamela Gay and Beth Johnson
Hosted by Pamela Gay and Beth Johnson
Audio and Video Editing by Ally Pelphrey
Content Editing by Beth Johnson
Intro and Outro music by Kevin MacLeod, https://incompetech.com/music/