Monday was the first day of the Lunar and Planetary Science Conference, and we are going to spend at least the next two weeks sharing as much science as possible. The conference is taking place virtually this year, and of course, Mars is the big focus. Plus icy worlds, volcanic worlds, and exoplanets, and we’re bringing you a little of everything.
Media
Transcript
Hello and welcome to the Daily Space. I am your host Dr. Pamela Gay.
And I am your host Beth Johnson.
And we are here to put science in your brain.
This week is the Lunar and Planetary Science Conference. Normally this meeting would bring together several thousand planetary scientists at a conference venue in Texas, but because of COVID times, this meeting is instead being held online, and we are here at the Daily Space are going to do the best we can to pour through the mix of live and pre-recorded science to bring you all the planetary news we can. But before we do that, we’re going to bring you news from the rest of the universe.
And our first story takes us out to the edge of the observable universe where a rare and brief event was spotted not once but three times. The event? Fast radio bursts (FRBs): millisecond long spikes in radio waves from distant objects, quite probably tiny dead stars with powerful magnetic fields called magnetars.
Because FRBs are so very brief, you have to be looking in exactly the right direction at exactly the right time with just the right equipment to be able to see them. And until now, just not that many have been spotted. Astronomers using the 500-meter FAST telescope in China are working to change that and are conducting a survey to systematically look for FRBs and determine their locations and how common they actually are.
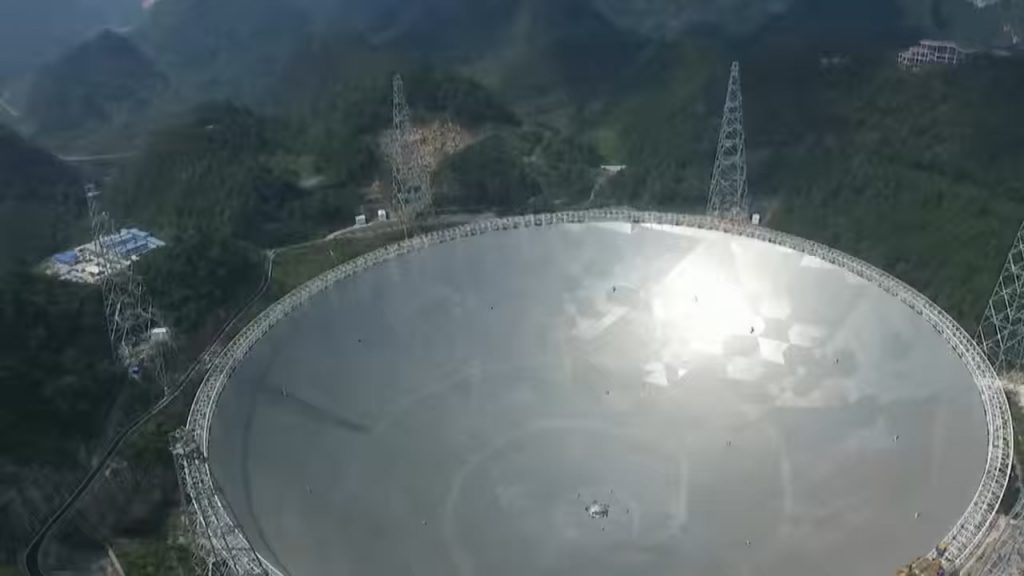
In a new paper in The Astrophysical Journal with first author Chen-Hui Niu, the team published three new FRBs located 4-5.5 billion light-years away. Based on how large an area they observed and how long they observed, this team estimates that if we could observe the entire sky with the right instruments, we’d detect 120,000 FRBs every day.
This survey is our latest recipient of the BABIES award for “Bad Acronyms & Backronyms in Everyday Science”. The survey’s name is CRAFTS which stands for Commensal Radio Astronomy FAST Survey and has an acronym nested in an acronym and randomly uses the first and last letters of that nested acronym. Well played, acronym creators. Well played.
While these kinds of surveys give us insight into the universe as a whole, to understand that universe, sometimes we also need to focus on understanding our own world, or in the case of this next story, the gravity of our world and the things in it.
In a remarkable new paper in the journal Nature, researchers from the University of Vienna show how they measured the gravitational pull of a 2-mm gold sphere weighing just 90 milligrams. This was accomplished by using lasers to carefully measure how the effects of the sphere’s gravity changed the swinging of a tiny pendulum of glass, four centimeters long and half a millimeter thick, capped with gold balls, and suspended from a glass fiber a few thousandths of a millimeter in diameter.
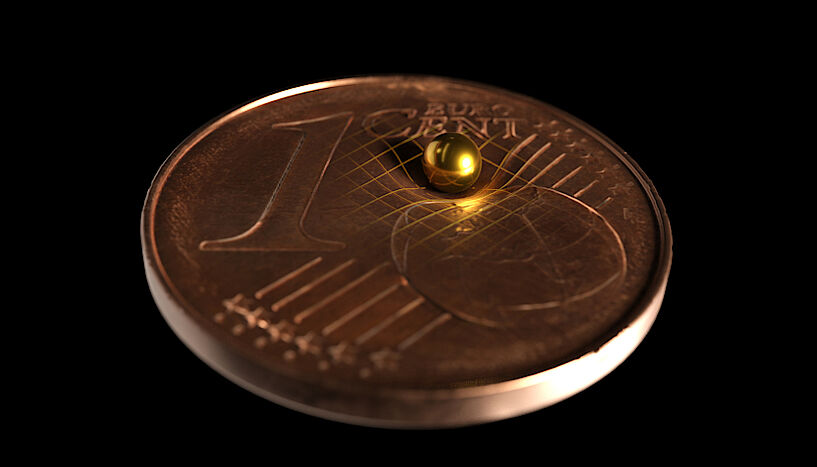
According to co-author Jeremias Pfaff: We move the gold sphere back and forth, creating a gravitational field that changes over time. This causes the torsion pendulum to oscillate at that particular excitation frequency.
These gold balls are roughly the mass of a ladybug! This experiment shows that it may one day be possible to measure traces of dark matter and dark energy by how they affect the gravitational field. Study lead author Tobias Westphal puts it this way: According to Einstein, the gravitational force is a consequence of the fact that masses bend spacetime in which other masses move. So what we are actually measuring here is, how a ladybug warps space-time.
And let’s face it, that is just kind of cool.
The changing gravitational force a pendulum, a person, or a detector is able to register is related to the changing locations of everything around us. This means that when a plane flies overhead, at the smallest level, your weight is reduced as you are pulled toward that plane. You won’t notice this; your bathroom scale can’t measure this, but the change in the gravitational field is real.
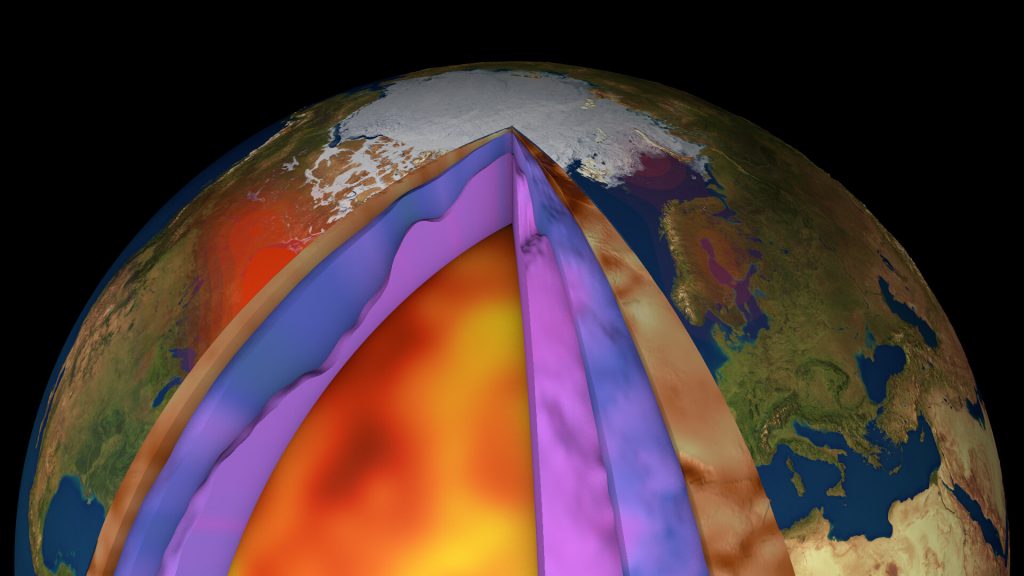
From 2009 to 2013, the ESA’s GOCE mission carefully measured the Earth’s gravitational field, and in a new analysis of the data, researchers have been able to measure the Earth’s crust and upper mantle. By better understanding these parts of the Earth, we can gain new insights into how the Earth’s plates move and initiate reactions like earthquakes. Using this data along with seismological observations and data on the properties of the crust, scientists have created a new model that is already helping us understand how the Earth continues to rebound from melted glaciers and the spreading rates of mid-ocean ridges. This work is published by J Dullea, S Lebedev, and NL Celli in Geophysical Journal International, and I look forward to seeing how this new model improves our understanding of our changing Earth.
It’s been a while since we talked about exoplanets around here. Oh, no… wait. No, it hasn’t. We always seem to be talking about exoplanets. And today I have three stories to share.
First up, distant planet GJ 1132 b is a sub-Neptune that lost its atmosphere due to being too close to its young, hot star and faced with intense radiation, making this sub-Neptune more Earth-sized. But wait! What’s this the Hubble Space Telescope has detected? An atmosphere? It seems that, in the face of losing its atmosphere, GJ 1132 b found a way to acquire a new one.

This secondary atmosphere was analyzed based on a combination of Hubble’s observations and some computer modeling. Instead of the primordial hydrogen/helium atmosphere, atmosphere 2.0 is made of hydrogen, hydrogen cyanide, methane, and an aerosol haze. The haze is likely photochemically produced hydrocarbons, similar to the smog that can form here on Earth.
Even more interesting, this new atmosphere has possibly been influenced by volcanic processes in the interior and on the surface of GJ 1132 b, and those processes are due to the tidal heating from the gravitational pull of the star and another planet, plus the elliptical orbit which gives those pulls some needed variation.
Lead author Mark Swain sums up the work, soon to be published in The Astronomical Journal: How many terrestrial planets don’t begin as terrestrials? Some may start as sub-Neptunes, and they become terrestrials through a mechanism that photo-evaporates the primordial atmosphere. This process works early in a planet’s life when the star is hotter. Then the star cools down and the planet’s just sitting there. So you’ve got this mechanism where you can cook off the atmosphere in the first 100 million years, and then things settle down. And if you can regenerate the atmosphere, maybe you can keep it.
Chalk another point up in the column for sub-Neptunes being able to shift to super-Earths, I guess.
Of course, much of current exoplanet research seems to have the goal of finding another habitable world. As I mentioned in the previous story, it seems that surface volcanism may be influencing the new atmosphere, and it turns out, it’s not just volcanoes that can have an effect. In new work published in The Planetary Science Journal, researchers determined that rocks and rock types can influence the composition of the atmosphere, which in turn leads to the habitability of the planet.
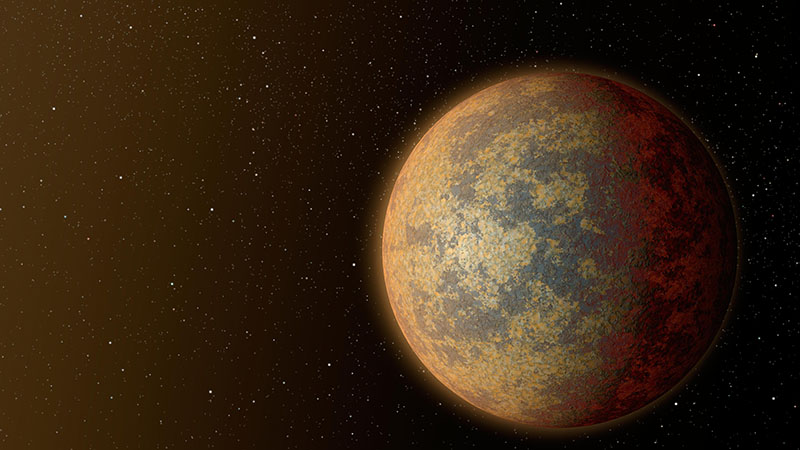
Lead author Kaustubh Hakim explains: We want to understand how the chemical reactions between the atmosphere and the surface of planets change the composition of the atmosphere. On Earth, this process – the weathering of silicate rocks assisted by water – helps to maintain a temperate climate over long periods of time. When the concentration of CO2 increases, temperatures also rise because of its greenhouse effect. Higher temperatures lead to more intense rainfall. Silicate weathering rates increase, which in turn reduce the CO2 concentration and subsequently lower the temperature.
Once again, this work was done through a lot of computer modeling, testing how various conditions affected the weathering process of rocks and how the results could impact any atmosphere. All of which helps us understand the probability of finding that first, truly habitable world.
From the atmosphere to the surface to the interior, this last story dives down into the mantles of rocky super-Earths. The work was published in Nature, and it involved lasers. No, seriously, lasers were involved. Big, powerful lasers at the Lawrence Livermore National Laboratory were used to compress iron oxide to pressures between three and five times that of the core-mantle boundary here on Earth. The compression took nanoseconds. Nanoseconds!
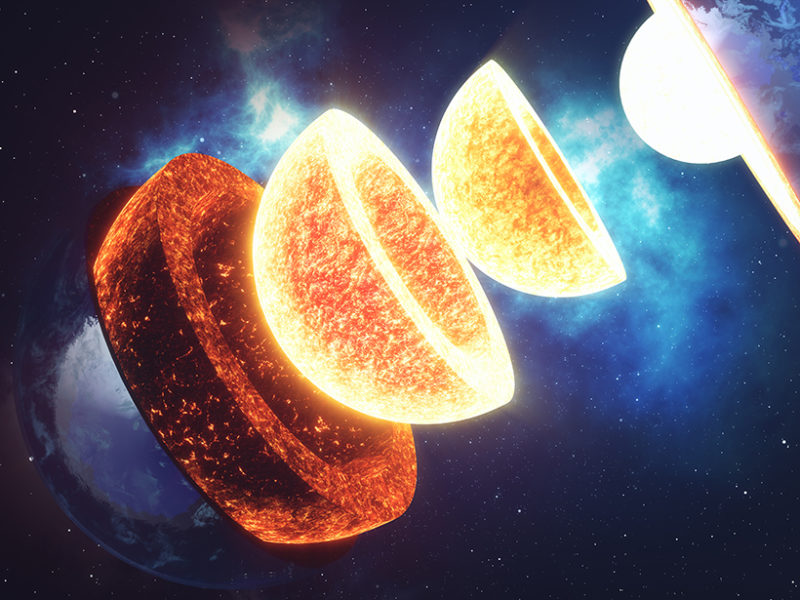
A little chemistry now. The iron oxide ended up at twice the density of another common mantle mineral, magnesium oxide, and it underwent a phase transition in the process. Here on Earth, you can easily mix the two minerals together in the mantle, but with that phase transition at high pressures, that’s no longer possible. This means that in a super-Earth, there could be some new physical properties we don’t see here on Earth.
Lead author Federica Coppari explains: Not only are the atoms more tightly packed, this new material phase [of iron oxide] is associated with a dramatic drop in viscosity…[which] plays an important role in the convecting motions inside the mantle. The rheology of a large extrasolar planet might be completely different than that of the Earth…and it’s related to the different material properties at more extreme conditions expected inside exoplanets.
This research shows a new way to experimentally derive some information about the characteristics of a type of planet we don’t have in our own solar system, and this is incredibly impressive to me. I know I joke a lot about planetary formation theories, but I genuinely love all the work being done, and again, this story involved lasers. Woo!
And now, let the LPSC firehose begin. This conference has presentations with all the latest news from around the solar system. This morning, the big show started with a deep dive into the landscape that the Perseverance rover now calls home.
While we have a pretty good idea roughly where a spacecraft should land, detailed mission planning can’t start until the exact landing spot is known. For just over twenty days, Percy has sat on the red planet, taking in its landscape with its amazing cameras, and while mission engineers do a complete check of the rover’s systems, team scientists have been planning their future journey through Jezero crater and out to Nili Planum beyond.
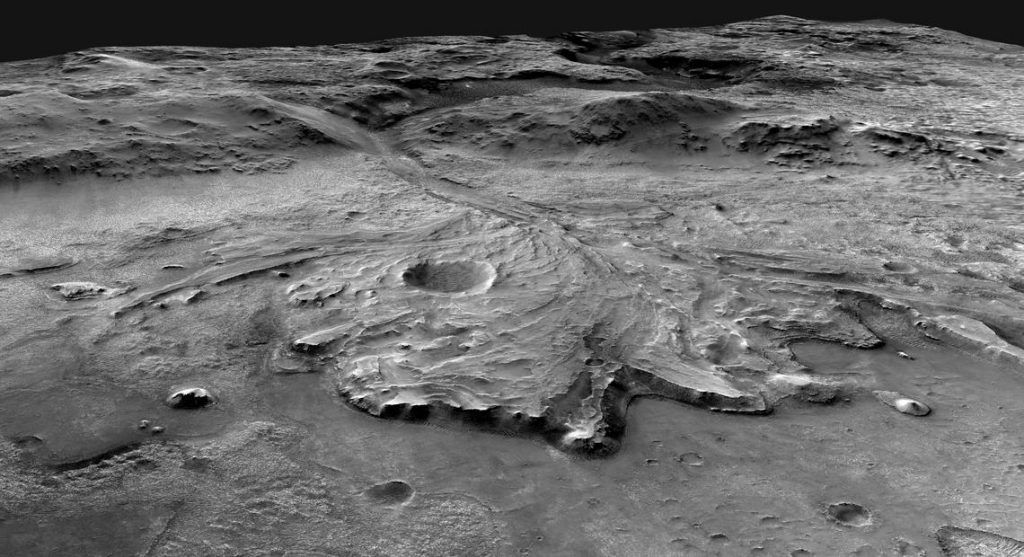
Jezero Crater is a much smaller geographical area than Curiosity’s Gale Crater. It is also a lot more hazardous and more interesting due to that dangerous and complex geology. Figuring out a path that keeps the mission safe and allows sampling of all the cool rocks is an awesome challenge, and to judge from Twitter, a whole lot of planetary scientists just want to run around wildly picking up rocks.
Making things complicated is a particularly dangerous section of land between the Octavia E. Butler landing site and where the rover most wants to go. Just like hikers on Earth, the mission is looking to just go around and simply needs to decide if they go around to the left or to the right before they move forward.
The mission team has plotted out a two-phase path that lets Percy, once it gets around that danger region, sample a variety of environments around a former lake, streams, and resulting deltas that shape Jezero Crater. They also look beyond Jezero to the Nili Planum to plan out this two-phase mission, during which the rover will collect samples in Jezero and leave behind one cache for future pick up and return to Earth, and to then rove through Nili Planum, a volcanic deposit, where during a hoped-for extended mission it will collect more rocks.
During Ken Williford’s presentation, he talked about how various features could hold minerals and organic materials deposited by past life. He literally compared existing formations on Mars to similar-looking structures on Earth created through the build-up of dead micro (and macro) organisms and plant life. The path he and others are planning is a tour of an ancient habitable environment where they are looking for the chemistry of life. We’ve seen these comparisons before, but it never stops making me catch my breath. Other speakers talked about this region as a dried-out aquifer and questions arose about where best to look for life, with Williford further commenting that “some believe that the Martian subsurface is the best bet for life now and also deep in the past.”
Yes, he said now. And yes, Perseverance is really well decontaminated, but that said, Percy isn’t going to go digging for life or go near places where life is likely today.
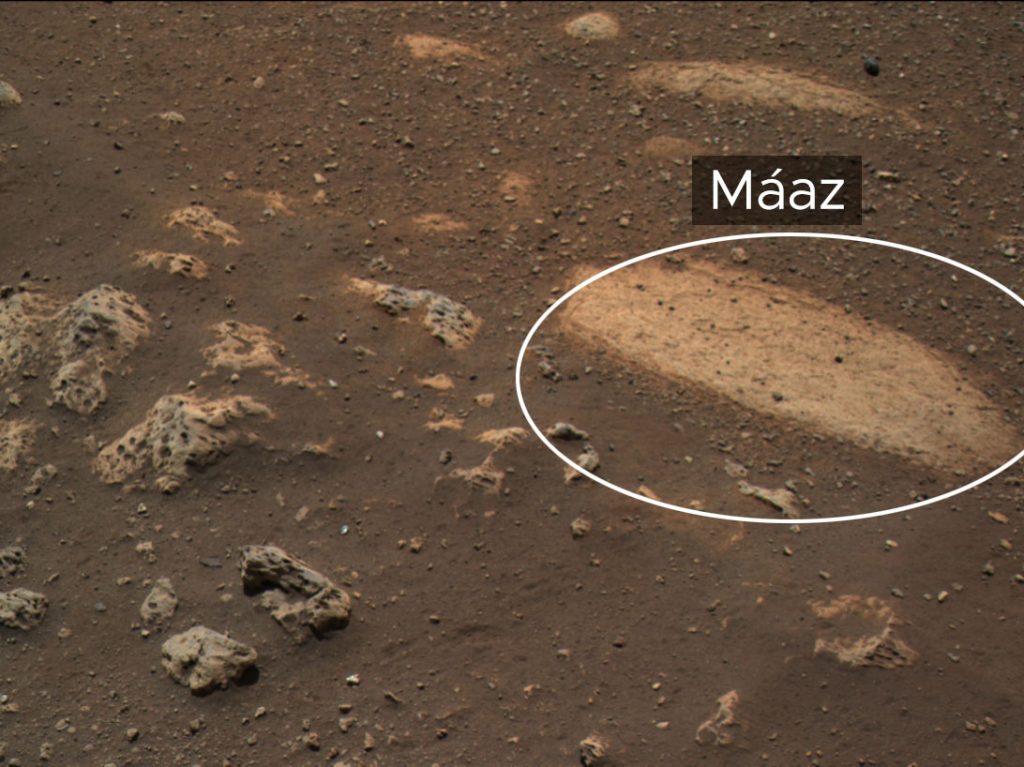
In Percy’s images, the landscape so far looks varied with dust, bare-baked mud-looking deposits, and a variety of rocks, including rocks that are hard to explain. My favorite of these are the holey rocks, which have been seen in the past but so far not sampled. These rocks look a lot like the Texas holey rocks loved by aquarium owners. The similar-looking rocks on Earth are made of limestone that has been shaped by water. I can only hope that Percy, with its arm and drill, will be able to reach out and finally tell us what these rocks are.
Not all talks at LPSC are about Mars. Yesterday, the highlight of the meeting was the exploration of all the places where oceans might exist beyond the Earth, and some of the oceans being discussed really made Earth feel like a dry desert world.
Let’s take a look at Pluto. Doctoral researcher Adeen Denton presented a new analysis of New Horizons images that hint at Pluto having a 150-km deep ocean beneath its icy crust. For comparison, the deepest point in the Earth’s ocean is the Challenger Deep in the Mariana Trench, which is just eleven kilometers deep.
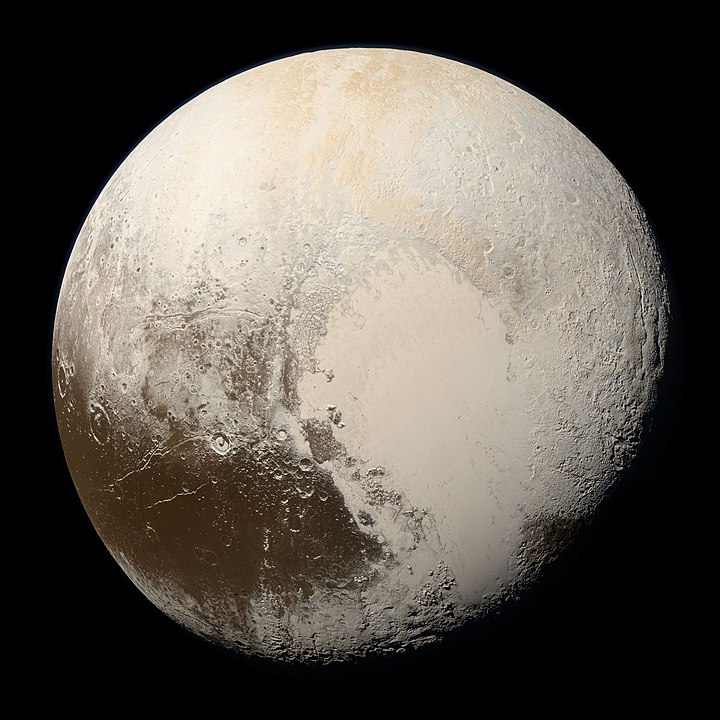
These results are thanks to a new analysis of Pluto’s low-resolution side. During the New Horizon’s flyby, the mission got extremely good images on one side of Pluto and lower resolution images of Pluto’s other side while the mission was further away. There are a lot of tricks of the trade that allow folks to eek more information out of images, and over time, as our software gets better, we’re able to reprocess things and learn more. Well, in reprocessing the low-res side of Pluto, they found a fascinatingly located region with a fascinatingly disrupted-looking terrain.
That location? Exactly opposite from Sputnik Planum, the smooth heart-shaped region imaged in such amazing detail. Many researchers have proposed that Sputnik Planum is actually an impact basin. If this is the case, when Pluto was hit by whatever it was hit by, the impact would have generated shockwaves that traveled through and around the planet. On other planets, we have seen a crater on one side, and a disrupted terrain on the antipode – the opposite side – where the shock waves came together and tossed the land around.
Different kinds of land, planetary core material, and stuff in-between can all transport these waves in different ways. Denton ran what she calls a “small army” of Pluto simulations that each had oceans of different thickness and cores of either serpentine or other materials that don’t matter because it was a core of serpentine and an ocean 150-km thick that produced the disruptions actually seen, we think, in the low-res images of Pluto.
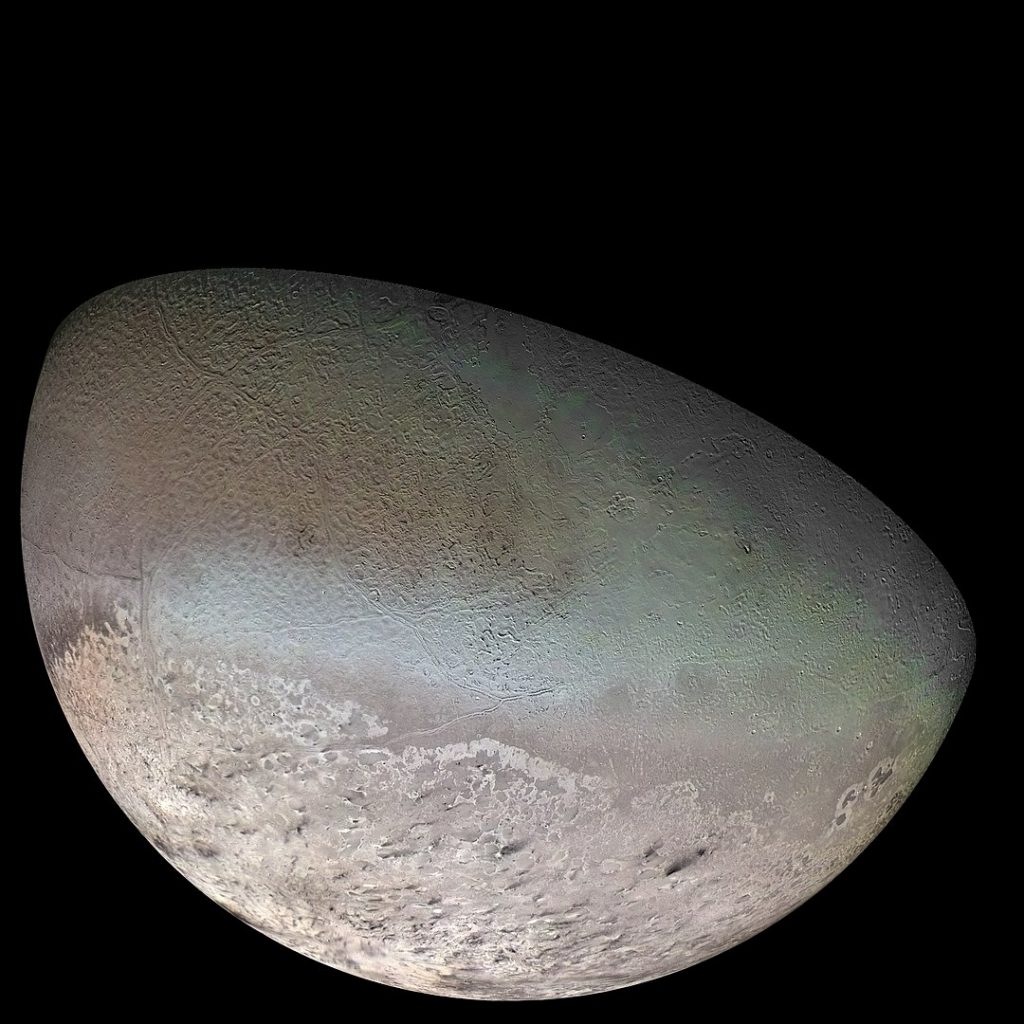
Understanding the interplay of water, rock, and heat was a recurring theme throughout this meeting, and when it comes to the interplay of heat and ice, melting is a common result. This comes into particular play with Neptune’s moon Triton. Larger than Pluto, this captured Kuiper Belt Object had to somehow drop a ton of kinetic energy as it went from orbiting the Sun to orbiting Neptune. While this can partially be explained if Triton, like Pluto, was in a binary system with another large body, that doesn’t explain everything.
Triton would have started in a highly elliptical orbit, going backward around Neptune, and that orbit would have become circularized over time through tidal effects that change the orbit while heating the world. Presented by Noah Hammond, new research finds that this process would have taken billions of years, and depending on the composition of Triton, potentially could have completely melted this world’s ice. In what was the sweetest analogy of the conference so far, researchers discussed how we can tell what is happening by comparing how the ice melted with how ice cream melts.
Specifically, think of how ice cream melts and spreads when placed on a hot pie. The top stays structured, but the entire surface spreads, and the edges change. All it will take to figure out if this is true is better images from a spacecraft. A mission named Trident is currently proposed, and if it is funded and makes it to Triton, its images of the meltiness will help us one day sort just what Triton is made of by looking at how it dissipated heat, like ice cream on a pie.
This has been the Daily Space.
Learn More
Three New Fast Radio Bursts Captured by FAST
- Chinese Academy of Sciences press release
- “CRAFTS for Fast Radio Bursts: Extending the Dispersion–Fluence Relation with New FRBs Detected by FAST,” Chen-Hui Niu et al., 2021 March 3, The Astrophysical Journal Letters
Warping Space-Time on a Very Small Scale
- Universität Wien press release
- “Measurement of gravitational coupling between millimetre-sized masses,” Tobias Westphal et al., 2021 March 10, Nature
Modeling Plate Tectonics with ESA’s GOCE Mission
- ESA press release
- “WINTERC-G: mapping the upper mantle thermochemical heterogeneity from coupled geophysical-petrological inversion of seismic waveforms, heat flow, surface elevation and gravity satellite data,” J Fullea et al., 2021 March 10, Geophysical Journal International
Rocky Sub-Neptune Exoplanet Found to be on Second Atmosphere
- NASA Goddard press release
- Hubble Telescope press release
- “Detection of an Atmosphere on a Rocky Exoplanet,” Mark R. Swain et al., to be published in The Astronomical Journal (preprint on arxiv.org)
Habitability of Exoplanets Influenced by Rocks
- University of Bern press release
- “Lithologic Controls on Silicate Weathering Regimes of Temperate Planets,” Kaustubh Hakim et al., 2021 March 11, The Planetary Science Journal (preprint on arxiv.org)
Understanding Super-Earths With Giant Lasers
- Eos article
- “Implications of the iron oxide phase transition on the interiors of rocky exoplanets,” F. Coppari et al., 2021 February 11, Nature Geoscience
LPSC 2021 Abstracts
- The Mars 2020 Mission One Month After Landing
- A Tour of Ancient Habitable Environments In and Around Jezero Crater, Mars
- Antipodal Terrains Produced by Sputnik Planitia-Forming Impact Imply Pluto Has a Thick Ocean and Hydrated Core
- Intense Geologic Activity on Triton for Billions of Years After Orbital Capture
Credits
Written by Pamela Gay and Beth Johnson
Hosted by Pamela Gay and Beth Johnson
Audio and Video Editing by Ally Pelphrey
Content Editing by Beth Johnson
Intro and Outro music by Kevin MacLeod, https://incompetech.com/music/