A new system has been found that consists of six stars in three binary pairs, which are producing a bevy of eclipses with each other. They were found in TESS data with the help of machine learning. Plus, Venus, more exoplanet news, difficulties with the record of early life, and a look back at Marie Tharp’s groundbreaking work.
Media
Transcript
Hello and welcome to the Daily Space. I am your host Dr. Pamela Gay.
And I am your host Beth Johnson.
And we are here to put science in your brain.
We don’t often have breaking news on this show, but after we’d set our show run for today’s episode, a story came out that made us drop everything, and make it our lead story. The story? The Transiting Exoplanet Survey Satellite, or TESS, has found a single star system with six stars, arranged in pairs, that, like dancers on a dance floor, all appear to circle in the same plane on the sky. Now, that may not sound that exciting initially, but the amount of things that have to go just right to get a system like this is pretty amazing.
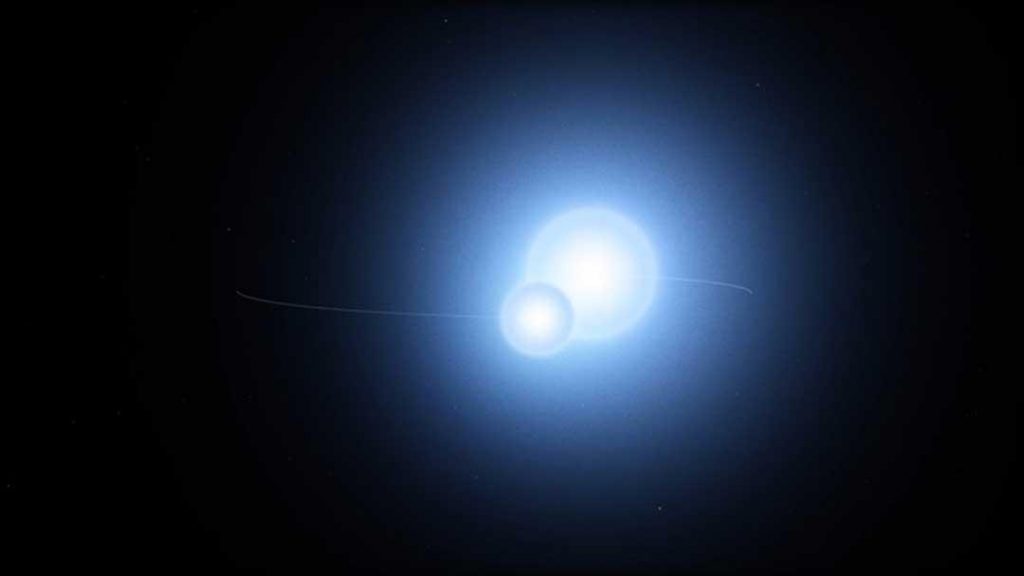
In a single telescopic image, all six of these stars appear as a single point of light that over time varies in brightness. That single point is cataloged as TIC 168789840. That single point, however, changes in brightness over time in a way that isn’t normal for a single star. This unusual pattern of dips was discovered by software designed to look for eclipsing binary stars in sets of images from TESS.
Eclipsing binaries are pairs of stars that are oriented just right in the sky so that the stars pass in front of and behind one another as seen from our perspective here on Earth. Stars can and do form in every possible orientation so that as we look across the night sky we see stars that eclipse, stars that spiral around each other, and everything in between.
In a completely random universe, only one in every several dozen binaries will be seen to eclipse, with the light we’re seeing getting fainter whenever we can see one of the stars hiding behind another. And this system… In this system, there are three pairs of stars that are precisely lined up to eclipse each other.
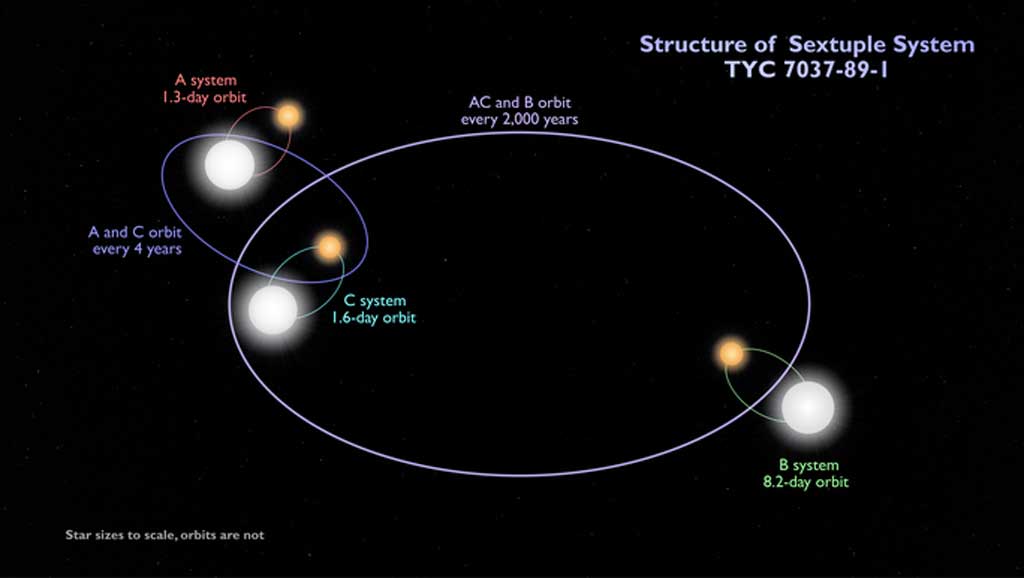
This isn’t the first six-star system we’ve discovered. If you’ve ever gone out and looked at the constellation Gemini and its bright star Castor, you’ve looked at a six-star system masquerading as a single star. This is, however, the first totally eclipsing system we’ve found, and part of what makes this so awesome is that this flat system hints at a star formation history that had all these stars forming out of a massive, flat, and spinning cloud of gas that broke into each of these stars rather than forming a single massive one. These aren’t large stars; they range from about half the size of our Sun to about 30% bigger than our Sun.
These pairs are arranged with two of the systems in a fairly tight orbit, and the third pair significantly farther away. It’s unlikely that planets capable of supporting life could orbit any of those four stars in the tight pair, but it might be possible to find habitable worlds associated with that third, farther out system — might. The data hasn’t shown any evidence so far, but this system is in need of a good science fiction writer or artist to image the skies and mythology of this kind of complex system.
It feels like Venus has been in the news a lot lately, which is surprising because up until last year, we mostly ignored our sister planet. The clouds are too thick, the surface is too hot, the air is too toxic, etc. Now we’re talking about sending more missions there than ever before, and the science coming out from a variety of work being done is being noticed by the press. Thank you, phosphine, whether or not you’re really there.
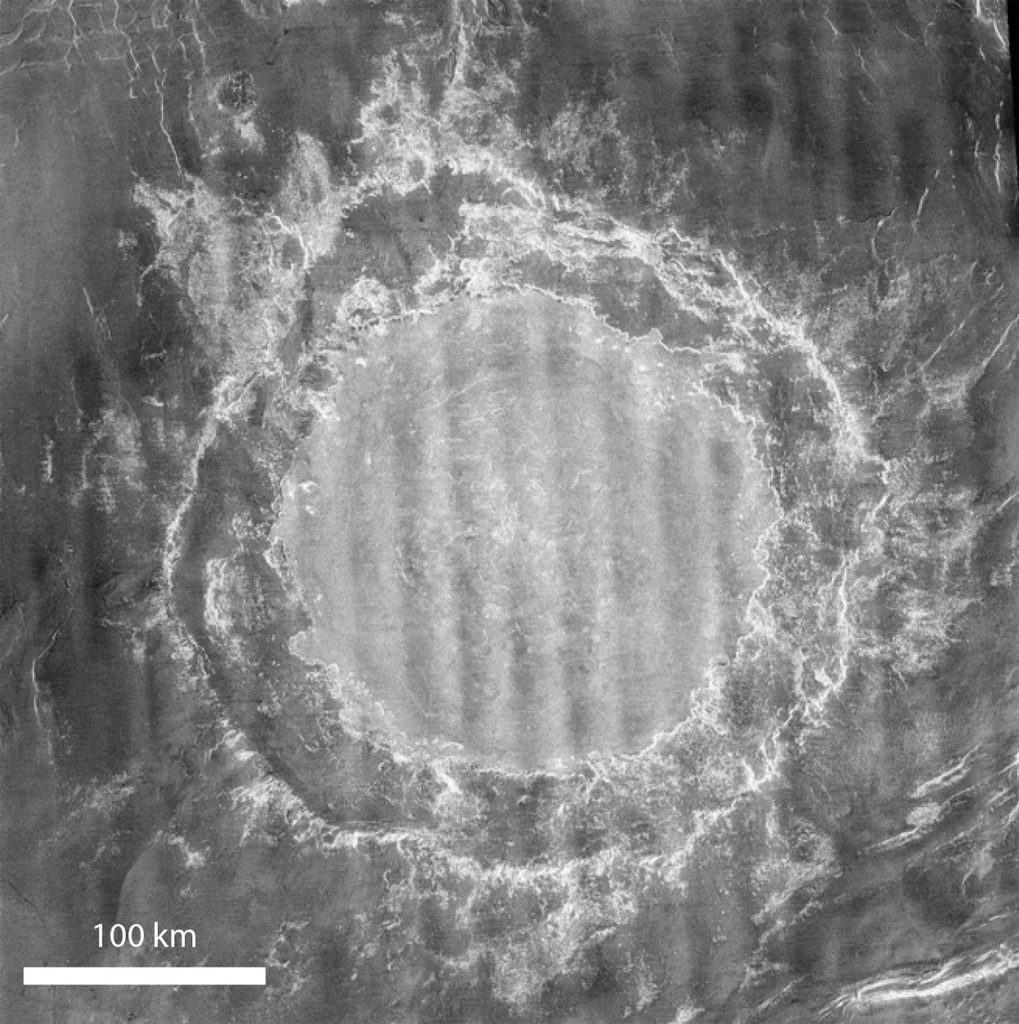
In our first planetary story today, a new paper published in Nature Astronomy uses the planet’s largest known crater to analyze the thickness of Venus’s lithosphere, that outer shell of crust. Here on Earth, our crust is considered thin. It is broken into plates that ride on the top of the mantle, which is warm and plastic. This configuration is why we have volcanoes and earthquakes — our planet is constantly changing, creating new land and destroying old crust.
Venus also has volcanoes. It is suspected they may even have been active in the recent geological past. But that thick atmosphere I mentioned earlier prevents us from directly imaging the surface to really analyze the rocks. In our interview with Dr. Darby Dyar last week, we talked about how there are spectroscopic windows future missions might be able to use to “see” that surface more clearly. In the meantime, we have to use the radio imaging data currently available to make sense of Venus. And one of the questions we’re trying to answer is whether or not Venus had a primitive form of plate tectonics that could account for those volcanoes.
Scientists used radio images of Mead Crater, the largest on Venus, to generate computer models that allowed them to analyze the thickness of the lithosphere. The crater is surrounded by two rings of ridges, and their final position is an indicator of the thickness and thermal gradient of the rocks that were impacted. Basically, it’s harder to deform colder rocks than warmer ones, and those characteristics determine the distance at which the impact rings formed.
What the team found was that the crust is thicker on Venus with a lower thermal gradient than on Earth and unlikely to have had similar plate tectonics. Graduate student and lead author Evan Bjonnes explains: You can think of it like a lake freezing in winter. The water at the surface reaches the freezing point first, while the water at depth is a little warmer. When that deeper water cools down to similar temperatures as the surface, you get a thicker ice sheet.
The Mead impact is about a billion years old, which means Venus hasn’t had active tectonics in at least that long, and that result changes what we thought happened in Venus’s past. We need those new missions to Venus, please.
The solar system we see today is seriously different from the one that formed four to five billion years ago. As we talked about a few days ago, trying to understand how solar systems formed is not something we’re really good at yet, and to get a good model that actually matches reality, we’re going to need to give theorists all the data we possibly can to help them out.
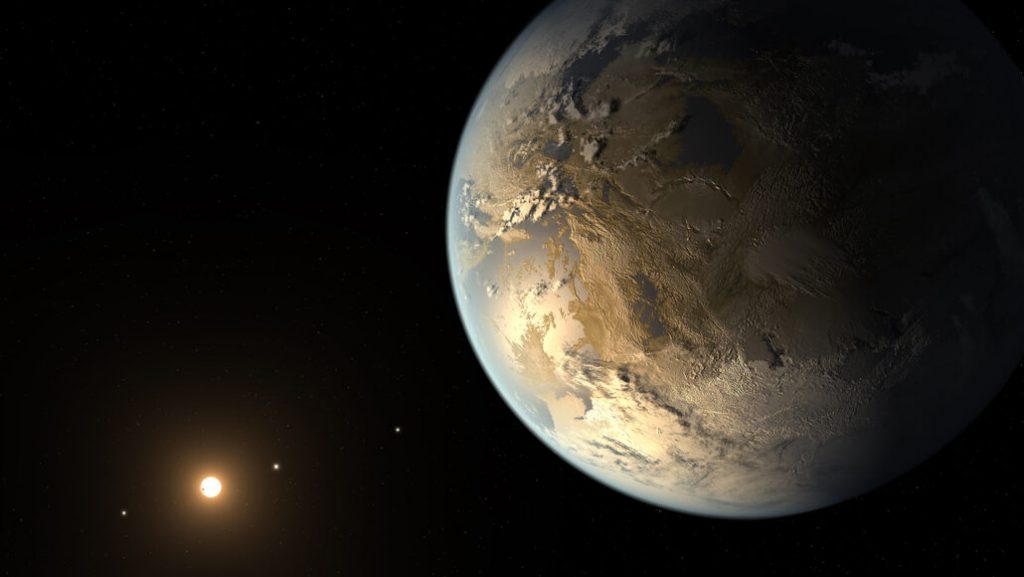
While we can’t go back in time and measure the conditions on early planets or sample the stuff the solar system was made of, the solar system did us a favor and set some samples aside to throw our way in modern times. These samples are the meteorites that periodically rain down on Earth and have trapped inside their stony exteriors samples of the gases and liquids — the volatiles — that made up our early solar system.
Researchers collect meteorites, and to get at these volatiles, they grind up and bake meteorite samples. A new study of three different meteorites, presented by Maggie Thompson at the American Astronomical Society meeting, suggests that our early solar system built rocky worlds rich in water and gases like carbon monoxide and dioxide, hydrogen, and hydrogen sulfide. They also found tiny amounts of some complex gases that haven’t yet been identified.
It may seem weird to bake meteorites to see what comes out, but this is pretty much what our Sun did to the early solar system, and that stuff that came out of the still-forming planets — that stuff became early planetary atmospheres. Over time, chemistry, geology — stuff like volcanoes and asteroid impacts — and even life modified planetary atmospheres in ways we can’t quantify. We don’t even know if bacterial or other simple life has existed anywhere except Earth!
Thanks to this and other studies of meteorites, we can see what was in the stuff that made the rocky worlds and start to understand how the atmospheres started. We can see how they ended. And hopefully, someday, science will figure out what happened in between.
Sometimes, a story isn’t so much about the science done but about who did the science. That’s the case with our next story. It’s about the discovery of yet another multi-planet system, which isn’t terribly exciting these days, except the discovery was made by a pair of high school students.
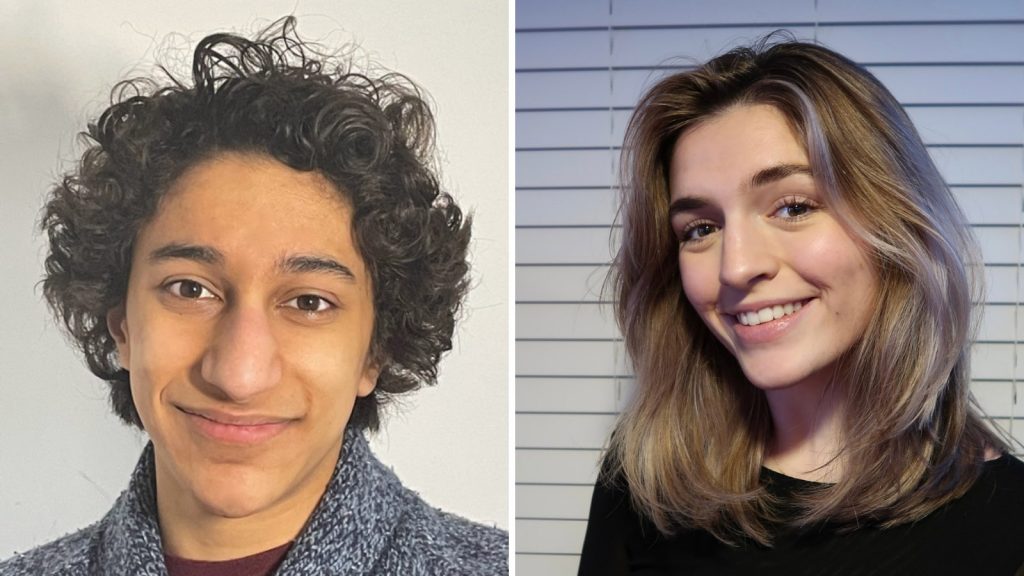
As part of the Science Research Mentoring Program (SRMP) run by Harvard and MIT, 16-year-old Kartik Pinglé and 18-year-old Jasmine Wright worked with postdoc Tansu Daylan to analyze data from NASA’s TESS. They looked at TOI-1233, which is a Sun-like star called HD 108236 about 200 light-years away from Earth, and they studied the dips in the light caused by potential exoplanets orbiting that star.
Much to their surprise and delight, they found four planets orbiting TOI-1233. Per the press release: Three of the planets are considered “sub-Neptunes,” gaseous planets that are smaller than, but similar to our own solar system’s Neptune. It takes between 6 and 19.5 days for each of them to orbit around TOI-1233. The fourth planet is labeled a “super-Earth” for its large size and rockiness; it orbits around the star in just under four days.
The results of this research were published this week in The Astronomical Journal with Pinglé and Wright as co-authors. The SRMP was founded in 2016, and provides about twelve students a year, mostly underrepresented minorities, with a paid research job. Pinglé is currently a junior in high school and contemplating majoring in applied mathematics or astrophysics. Wright was recently accepted into a five-year astrophysics master’s program in Scotland. Congratulations to you both and good luck in your future careers. We expect big things.
We are about two weeks away from NASA’s Perseverance Rover landing on Mars in Jezero Crater. This particular site was picked because it appears, in satellite imagery, to be a former lake bed, and is just the kind of place where, if Mars had life, we might expect to find fossils.
Yes, folks, Percy is going fossil hunting.
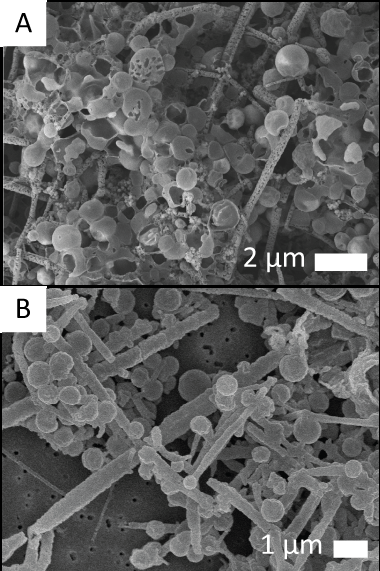
The problem is, even if Percy finds what appears to be fossilized bacteria, it will be hard to verify that what it sees is evidence of life and not a look-alike chemical reaction. Just how hard it will be was brought home by a new research study published in the journal Geology. Experiments by geobiologists Julie Cosmidis, Christine Nims, and their colleagues found that random chemical reactions left in the residue of their lab equipment closely resemble bacteria, and these structures can be fossilized very easily.
According to Nims: …under the microscope, you could see these beautiful structures that looked microbial. And they formed in these very sterile conditions, so these stunning features essentially came out of nothing. It was really exciting work.
Cosmidis goes on to explain: We thought, ‘What if they could form in a natural environment? What if they could be preserved in rocks?’ We had to try that, to see if they can be fossilized.
The team called these structures biomorphs — shapes that look biological. They simulated the formation of silica-rich rocks with fossils by carefully mixing silica solution and the biomorphs under conditions that mimicked those found when fossils form. According to a release related to this paper: They found not only that they could be fossilized, but also that these [biomorphs] were much easier to preserve than bacterial remains. The [biomorphs] ‘fossils,’ structures … were more resilient and less likely to flatten out than their fragile biological counterparts.
Cosmidis puts it this way: Microbes don’t have bones. They don’t have skins or skeletons. They’re just squishy organic matter. So to preserve them, you have to have very specific conditions, so it’s kind of rare when that happens.
If biomorphs can be created by accident in the residue in labware and easily fossilized, this raises the disturbing possibility that we are going to find a whole lot of fossilized biomorphs, which are not life, in the mix with whatever extremely rare, actually fossilized bacteria may be out there.
We already knew this could be a problem. About twenty years ago, researchers found what they said could be fossilized nano-bacteria in a Mars meteorite, and in the months and years after their announcement, teams the world over have found ways to explain their results as anything except life.
I personally am hopeful that Percy will find something fairly uncontroversial. I’m wishing for a nice, beautiful collection of stromatolites — fossilized bacterial mats like we see here on Earth. To be fair, anything we find will have folks arguing, “That can’t be fossilized life because…”, but if we can show that life here is similar to what began out there, far across the solar system, it will give us a new understanding of how life might fight to survive out there beyond the heavens.
Many of our listeners learned about plate tectonics in grade school. It’s an accepted theory, which means we have found tons of evidence for its existence, even if we can’t physically pull apart the Earth and see the plates and their layers directly. We’ve built up a veritable mountain of data with fossil records, seafloor spreading, earthquakes, and volcanoes. It’s a standard in geology lessons.
But it wasn’t always this way. In fact, some of you might be surprised to learn that plate tectonics wasn’t scientifically accepted by much of the geology community until the late 1960s, and it was proposed by Alfred Wegener back in the early 1900s. He proposed a hypothesis of continental drift and named a previous supercontinent Pangaea. And he was widely criticized and considered a bit of a crank, but his work started a debate in the field that stagnated with little evidence in his favor.
That all changed in the 1950s when the advancement of sonar technology during World War II led to large-scale mapping of the Atlantic Ocean’s seafloor. Enter Marie Tharp, one of my heroes, who was in the right place and the right field at the right time to advance the cause of continental drift in a big way.
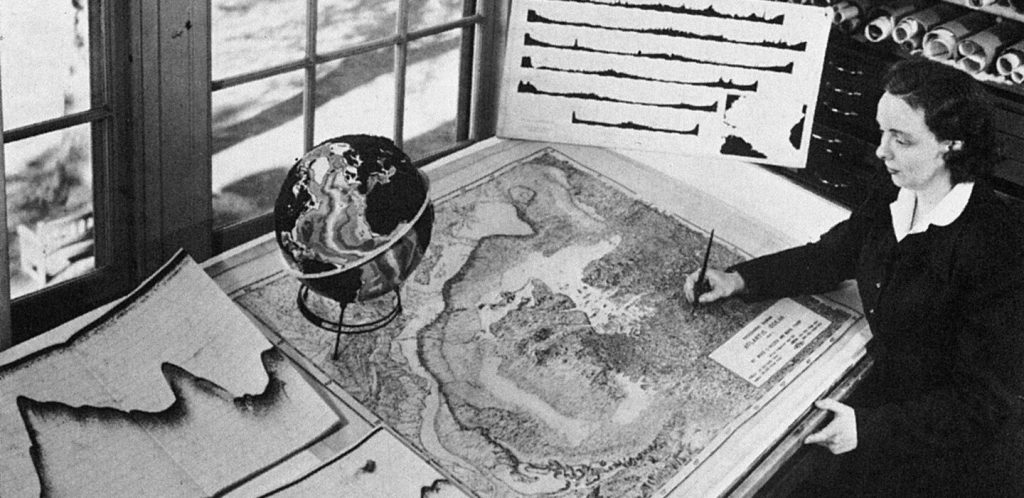
Marie Tharp was born in 1920. Her father was a surveyor who made maps, and she frequently went out into the field with him. In 1941, she was attending Ohio University, and Pearl Harbor was attacked, bringing the United States into World War II and essentially removing all the young men from the workforce. To keep programs up and running, many university departments opened their doors up to women, finally, and Tharp jumped at the chance to join the geology department. She earned her first master’s degree in 1943 and the second one in mathematics from the University of Tulsa several years later.
Tharp loved fieldwork, but it wasn’t open to women, especially on the open seas, where all of this new sonar scanning was being done. She moved to New York and took a job at Columbia University as a “draftsperson assisting male graduate students working toward a degree in geology that she had already earned.” It was a job, and it was in her field. When Columbia founded the Lamont Geological Observatory, Tharp was offered a position working with geologist Bruce Heezen, who worked with all that wonderful oceanic data coming in. Tharp was his analyst and mapper.
That’s where this story goes from good to amazing. Tharp was analyzing the data from scanning strips done of the Atlantic Ocean, and she noticed something interesting. Not only was there a ridge running down the middle of the ocean, which people had noticed in the years prior, but there was a notch at the top of the ridge in each profile. Tharp thought this might be evidence of molten material coming up from below the crust and forming new land; evidence that could support this wild theory of continental drift that was still being debated.
By 1957, Tharp and Heezen had created an extensive physiographic map of the Atlantic basin, clearly showing the mid-Atlantic ridge. At the same time, Heezen was collecting data to help Bell Labs lay transatlantic cables, and he found that earthquakes clustered along the rift valleys Tharp had found. The combination of the maps and the earthquakes helped solidify the idea that the crust was being pulled apart along the ridge.
Around the time the theory of plate tectonics finally was accepted, Tharp and Heezen were asked to work with Austrian painter Heinrich Berann on ocean illustrations. They produced maps that were published in National Geographic as huge supplements between 1967 and 1971. Then in 1973, the U.S. Navy gave them a grant to do the same for the oceans around the globe, and that work produced the map seen at our website, DailySpace.org, which was finished in 1977 [Ed note: The map, not the website].
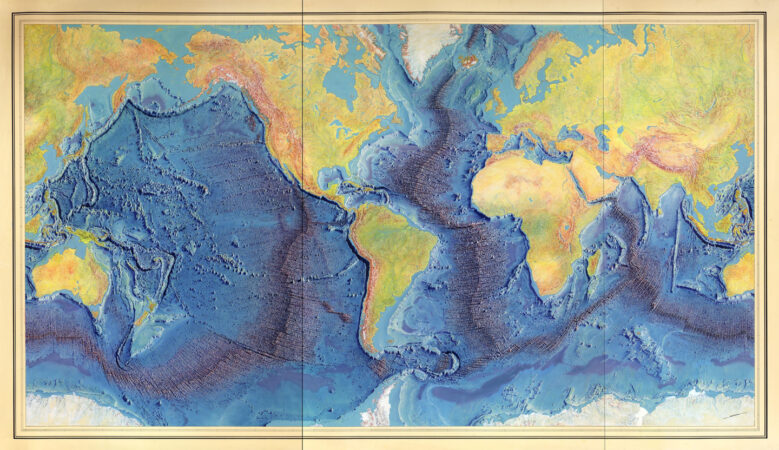
Unfortunately, Heezen died suddenly of a heart attack while in a submarine exploring the ridge near Iceland, and Tharp’s work dried up almost immediately. Like most women of her time, she went uncredited for a lot of the papers her data was included in, although her name is on the maps she helped produce.
Tharp received numerous awards later on in life, in recognition of her contributions to the fields of geology and mapping and plate tectonics. She died of cancer in 2006, but by all accounts, she was incredibly satisfied with the life she led. In fact, she said in 1999: I thought I was lucky to have a job that was so interesting. Establishing the rift valley and the mid-ocean ridge that went all the way around the world for 40,000 miles — that was something important… You can’t find anything bigger than that, at least on this planet.
Thank you, Marie Tharp, for your amazing work and your inspiration.
This has been the Daily Space.
Learn More
Sextuple Eclipsing Binary System Found in TESS Data
- SETI Institute press release
- NASA Goddard press release
- “TIC 168789840: A Sextuply-Eclipsing Sextuple Star System,” Brian P. Powell et al., to be published in The Astronomical Journal (preprint on arxiv.org)
Plate Tectonics Unlikely in Recent Past for Venus
- Brown University press release
- “Estimating Venusian thermal conditions using multiring basin morphology,” E. Bjonnes, B. C. Johnson and A. J. Evans, 2021 January 28, Nature Astronomy
Pulverized Meteorites Help Analysis of Early Atmosphere
- Science News article
High School Students Discover Multi-planet System
- Center for Astrophysics | Harvard & Smithsonian press release
- “TESS Discovery of a Super-Earth and Three Sub-Neptunes Hosted by the Bright, Sun-like Star HD 108236,” Tansu Daylan et al., 2021 January 25, The Astronomical Journal
The Problem with False Positives in Search for Early Life
- GSA press release
- “Organic biomorphs may be better preserved than microorganisms in early Earth sediments,” Christine Nims et al., 2021 January 27, Geology
Marie Tharp: A Retrospective
- Science News article (Marie Tharp)
- Science News article (Theory of Plate Tectonics)
Credits
Written by Pamela Gay and Beth Johnson
Hosted by Pamela Gay and Beth Johnson
Audio and Video Editing by Ally Pelphrey
Content Editing by Beth Johnson
Intro and Outro music by Kevin MacLeod, https://incompetech.com/music/
Cool.
I forwarded a link to this story to others at the Scientific Visualization Studio. Some of our earlier Earth science visualizations used the classic bathymetry whose origin you describe here.
Plus I grew up with that depiction in many of the world atlases I had in the 60s-70s.