Media
Summary
It’s a day ending in ‘y’, and planetary formation theories are once again being challenged. This time the challenge comes from a six-planet system with five planets in resonance. Plus a cloudless Jupiter, TRAPPIST-1, volcanoes, and some science of the weird.
Transcript
Hello and welcome to the Daily Space. I am your host Dr. Pamela Gay.
And I am your host Beth Johnson.
And we are here to put science in your brain.
There’s a volcano in Russia. Well, there are a lot of volcanoes in Russia, out on the Kamchatka peninsula, across the Bering Sea from Alaska, right on the ring of fire. In fact, Kamchatka is kind of known for its volcanoes and not a whole lot else it seems.
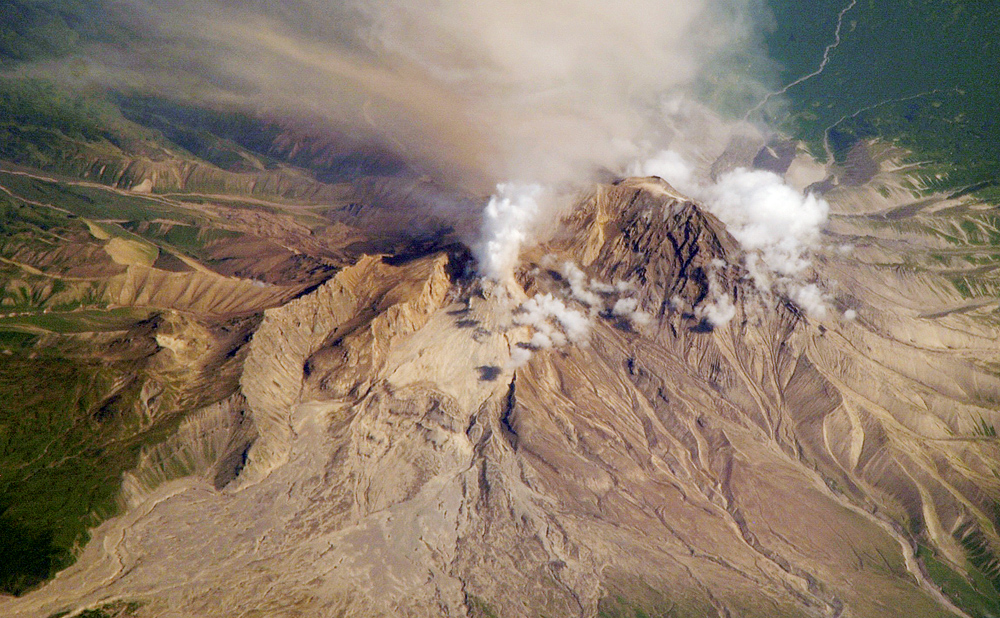
Those volcanoes are incredibly active. After all, the Pacific plate is subducting under the North American plate along the peninsula, causing an arc of volcanic activity.
Wait, Beth. Did you say “under the North American plate”? Yes, yes I did. The North American plate goes all the way around the Bering Sea. That part of Russia is on the same plate as we are. It’s a bit mind-boggling.
Anyway, the volcanoes on the peninsula are very active. There are thirty active volcanoes in all, and at the current moment, eight of them are erupting, including the one involved in our top story today: Shiveluch. Shiveluch has had over forty violent eruptions in the past 10,000 years, and while the last major blast was in 1964, it has been constantly erupting for more than 20 years now. It’s considered the most explosive volcano in the world and scientists want to understand why, so a team set about to collect what we call “primitive magma” that had been preserved as small nodules in larger bits of rock that had erupted. Kids, do not try this at home.
Primitive magma is magma that basically crystallized underground a long time ago and didn’t undergo the chemical changes that occurred later in a volcano’s life. So the volatile content — all that pressurized gas and liquid — is frozen in time and can tell us about the past conditions underground. If we can figure out what those past conditions were, we might understand what changes happened and use that to predict how other volcanoes may change over time.
Seriously, it’s amazing what you can learn from tiny crystals in rocks.
The team analyzed the minerals in the nodules and found that the water content by weight was in the 10-14% range. Eight percent water is considered super hydrous for subduction zone volcanoes, and 4% is considered average. These results were pretty striking and mean there must have been a lot of water underneath Shiveluch, more so than expected from a magma pool being hydrated by subducted wet rocks. It also means that the magma was at a lower temperature than usual.
As co-author Michael Krawczynski explained: The only way to get primitive, pristine materials at low temperatures is to add lots and lots of water. Adding water to rock has the same effect as adding salt to ice; you’re lowering the melting point. In this case, there is so much water that the temperature is reduced to a point where amphiboles can crystallize.
Amphiboles are a set of minerals that form at known pressures and temperatures as magma cools, and they are considered hydrated minerals due to their hydroxide content.
These results were published in the journal Contributions to Mineralogy and Petrology and could help us understand how the global water cycle works and possibly even give us some insight into the plumbing systems of other volcanoes.
And now is the time on The Daily Space where we share all that’s new in exoplanets and planetary formation theories, which just won’t settle down already.
We start with the star TOI-178, about 200 light-years away. When scientists first observed this star, they initially thought it had two planets in orbit at the same distance, which is, of course, unheard of. Yes, we have Lagrange points, but those tend to collect small rocks, not planet-sized masses.
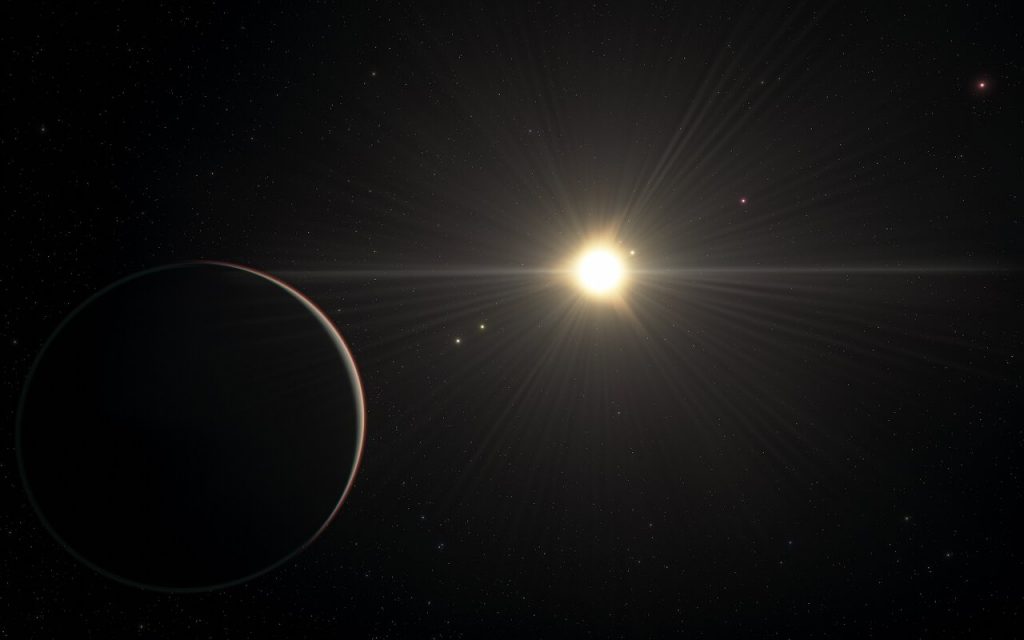
Further observations and analysis revealed that this star has not two, not three, but six planets in orbit around it. And it gets cooler and simultaneously weirder from there. Five of those six planets are in resonance around the star.
We see resonance in our own solar system, and I’ve talked about it before with respect to three of Jupiter’s Galilean moons. This resonance is where Io orbits Jupiter four times for every two orbits of Europa and every single orbit of Ganymede. It’s like a three-part harmony, and we call it a 4:2:1 resonance. Makes sense so far.
We’ve seen resonance in quite a few exoplanetary systems, ranging from two to four planets. And we’ve seen even more systems that are in a near resonance, where they’re really close to something like a 4:2:1 ratio but it’s off by a couple of percent either way for each planet. However, this system presents the first time we’ve seen a perfect resonance chain with five planets, and their ratio turns out to be an 18:9:6:4:3 chain.
The innermost planet of this chain is the second one from the star, and it goes around eighteen times for every three times the outermost planet orbits, and it does it in a couple of days. The results will be published in the journal Astronomy & Astrophysics, and co-author Yann Alibert explains further: The orbits in this system are very well ordered, which tells us that this system has evolved quite gently since its birth.
But then the system gets even more strange, as the compositions of these planets make no sense based on what we know of solar system formation. And I remind you that what we know keeps turning out to be less than we think. Co-author Nathan Hara describes the system: It appears there is a planet as dense as the Earth right next to a very fluffy planet with half the density of Neptune, followed by a planet with the density of Neptune. It is not what we are used to.
Once again, a new planetary system has challenged what we think we know. Must be a day ending in ‘y’.
It doesn’t even take an entire planetary system to challenge our knowledge of planetary formation. Sometimes, all you need is a strange planet. In the case of our next story, that strange planet is WASP-62b, which was discovered back in 2012 but didn’t become graduate student Munazza Alam’s project until recently. Alam is doing her thesis on exoplanet characterization, so she looks at known exoplanets and analyzes their atmospheres.
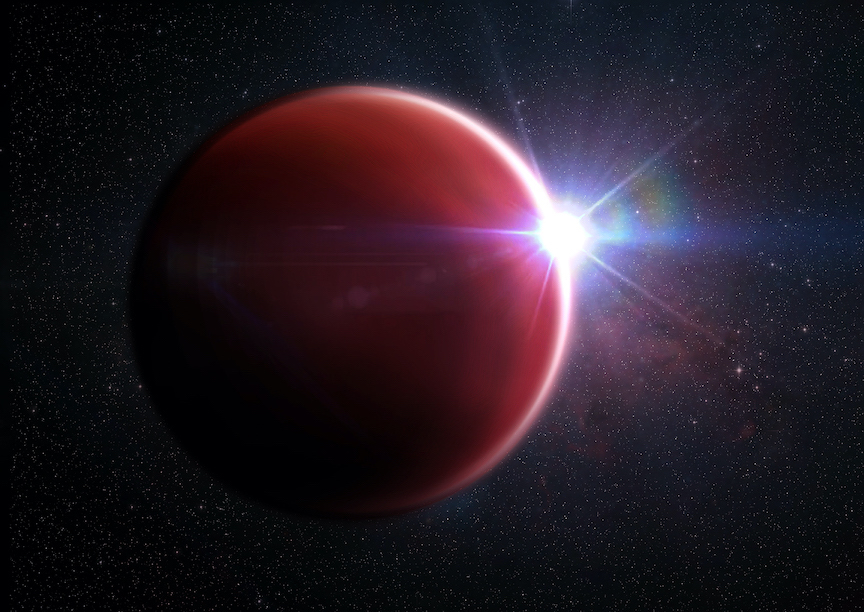
Enter WASP-62b, a hot Jupiter about 575 light-years away and half the mass of our own Jupiter that only takes 4.5 days to orbit its star. It’s extremely hot as a result, and since we have an abundance of hot Jupiters in our exoplanet catalog, likely ignored for that reason as well. In fact, Alam said: I’ll admit that at first, I wasn’t too excited about this planet. But once I started to take a look at the data, I got excited.
The data were spectroscopic observations done with the Hubble Space Telescope, and they showed that sodium was present. Not only was it present, but the absorption lines were complete, meaning no clouds in the atmosphere were present to block the sodium signature. Since less than 7% of studied exoplanets have a clear atmosphere like WASP-62b, this result was surprising. Alam notes that the rarity “suggests something else is going on or they formed in a different way than most planets.”
Of course, with no clouds, we might be able to use JWST to study the composition of the planet itself, and that might help with our pesky planetary formation hypotheses.
In more interesting exoplanet news that solves basically nothing, there is this fascinating system some of you may have heard of a time or two — TRAPPIST-1. This system was discovered in 2016 and made headlines for having seven planets, all similar in size to Earth, and quite a few of them in their star’s habitable zone. That made the system an excellent target for planetary scientists and SETI researchers alike.
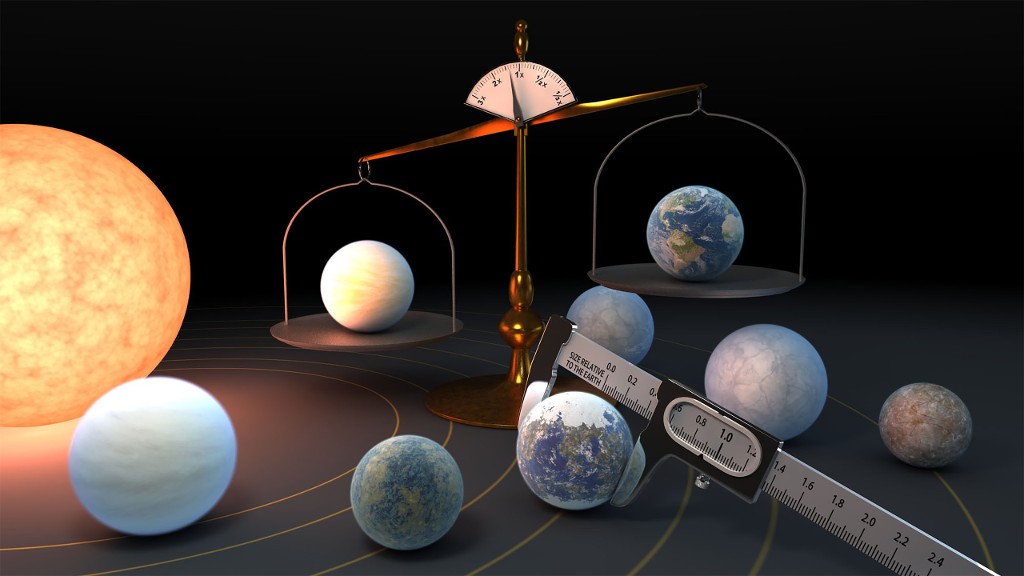
The planetary scientists got tons of telescope time on Kepler and Spitzer in space as well as TRAPPIST and SPECULOOS on the ground. Hours and hours of observations were done, and now a new paper published in The Planetary Science Journal looks at the results surrounding the densities of these seven worlds.
Co-author and doctoral student Elsa Ducrot explains: Our new study has greatly improved the precision of the densities of the planets, the measurements obtained indicating very similar compositions for these seven worlds. This could mean that they contain roughly the same proportion of materials that make up most rocky planets, such as iron, oxygen, magnesium, and silicon, which make up our planet.
The density of all these worlds turned out to be about 8% less than Earth, and the team came up with three hypotheses on why that is. One hypothesis keeps the composition of the planets similar to Earth but with 21% iron instead of 32%, which means the core is iron-depleted and lower mass than Earth’s. The second hypothesis uses rust as the reason for a lower iron content; the planets have vastly more oxygen than Earth, and that oxygen has oxidized the iron all the way through to the core. It’s even possible that the density difference is a combination of the two hypotheses.
Finally, the third hypothesis gives the planets a water content of 5% of the total mass in the outer planets of TRAPPIST-1. These planets are far enough out to still have liquid water, while the inner three planets would either have lost their water completely due to the star’s heat or had Venus-like atmospheres to prevent the water vapor from escaping.
Co-author Caroline Dorn sums up the work: The TRAPPIST-1 system is fascinating because around this unique star we can learn about the diversity of rocky planets within a single system. And we can also learn more about a planet by studying its neighbors, so this system is perfect for that.
Clearly, more work needs to be done to understand this unusual system. Or any system.
One of my favorite things about the universe is that, like a well-trained improv actor, it’s response always seems to be “Yes, and”.
In putting together today’s episode, I learned about a new way to form galaxies. In grad school, we were taught that galaxies either formed through the bulk collapse of material into massive systems or through the collapse of material into smaller systems that merged into bigger systems over time. In recent years, we’ve learned there is no “or”. Both these things are happening and it is awesome.
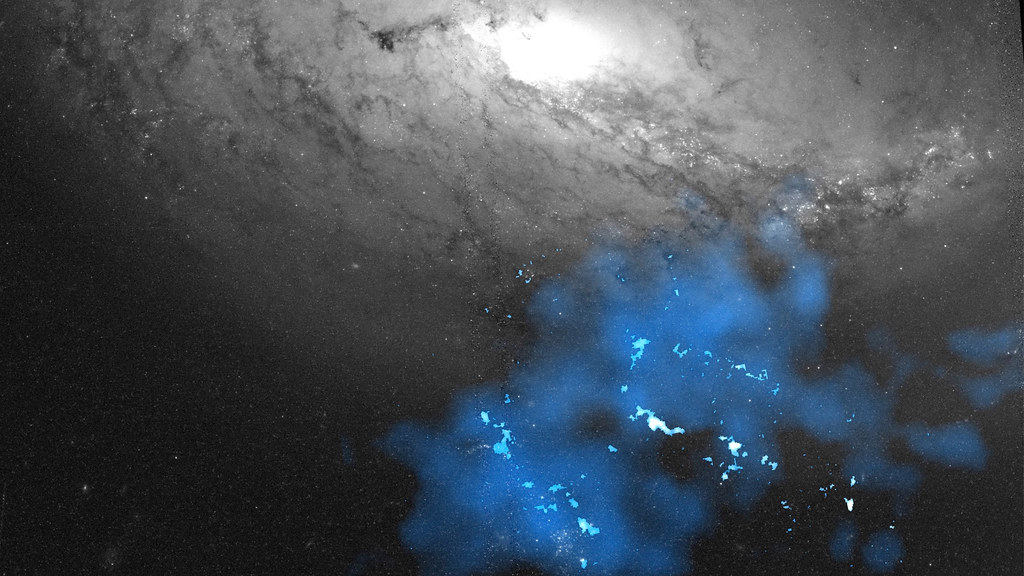
Today I learned, there is yet another possible way to get galaxies! During the massive galaxy collisions, streams of material can get torn out of the merging systems, and this debris can form entirely new galaxies, called tidal dwarf galaxies, or TDGs. These are new systems made of old material and are capable of star formation. In a new paper in Astronomy & Astrophysics, a team led by Miguel Querejeta, researchers use ALMA to study TDG J1023+1952.
According to co-author Carole Mundell: The little galaxy we’ve been studying was born in a violent, gas-rich galactic collision and offers us a unique laboratory to study the physics of star formation in extreme environments.
The star formation they found wasn’t entirely like we’re used to seeing in our galaxy. Here, our gas is generally tied up into giant molecular clouds that can collapse into star-forming regions. In this tidal dwarf galaxy, they also found diffuse gas throughout the system, and that gas is somehow forming stars.
And this is another set of “ands”: galaxies form through the collapse of material, they form through mergers, and they form from the material discarded in mergers. Stars form in giant molecular clouds, and they can form in diffuse gas permeating these special systems.
The science we had was all on track – it just lacked the creativity needed to anticipate all the crazy improv our universe seems ready to do.
This “yes, and” with galaxy mergers was a recurring theme in today’s galaxy papers. In a different paper, appearing in Nature Astronomy and led by Yohei Miki, researchers modeled what could happen during a variety of different kinds of galaxy mergers, with systems of many different sizes colliding in a variety of different ways. This time the focus was on how gas and dust in the systems moved, and if it would flow toward the galaxy’s central massive black holes, or instead get pushed elsewhere.
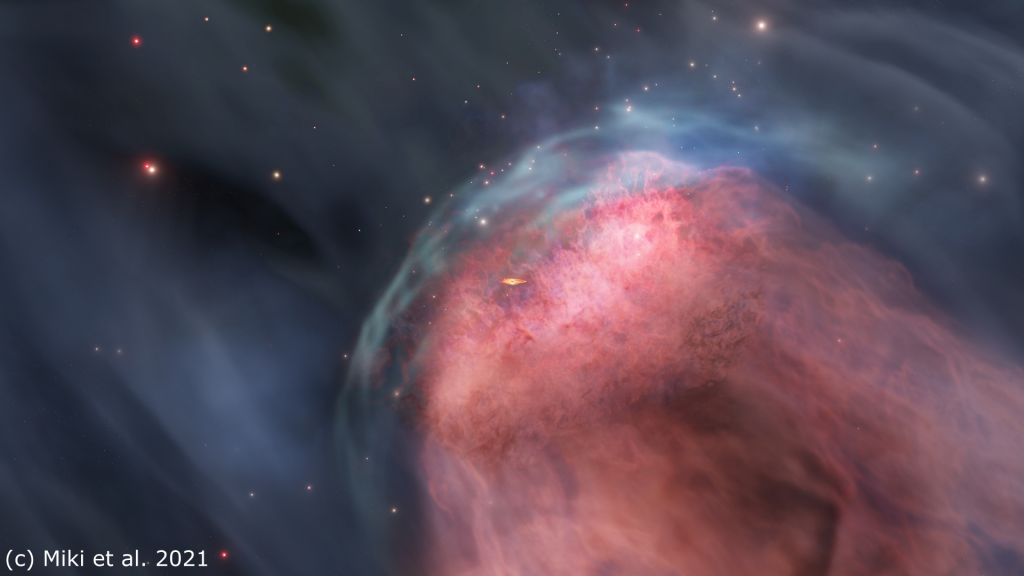
We know that some galaxy mergers do lead to material falling toward the galaxy centers where it can form a bright disk around the supermassive black hole as it falls in. We can see these systems; we know they’re out there. The open question is: does it have to be this way? Do all mergers lead to black holes getting large meals?
It turns out, if a small galaxy hits a larger galaxy just right, it can actually starve the black hole. Essentially, the interaction shapes the material around the supermassive black hole into a giant donut that the supermassive black hole can’t munch on. More scientifically, that donut shape is called a torus, and according to Miki: If the incoming galaxy accelerated this torus above a certain threshold determined by properties of the massive black hole, then the matter would be ejected and the massive black hole would be starved. These events can last in the region of a million years, though we are still unsure about how long the suppression of massive black hole activity may last.”
No matter how long it lasts, this is cool science and may even help us understand our own galaxy’s quiet past. We know we’ve merged with lots of smaller systems and we see evidence of past black hole activity. While we can’t go back and see what happened, these simulations may be giving us a hint at our history.
One of the more annoying limiting factors in doing a lot of science is our atmosphere. Place-to-place variations in temperature, humidity, and other factors lead to the twinkling of stars that we enjoy as children and hate as astronomers.
New technology is allowing us to overcome this twinkling in ever more impressive ways, and a recent test of a laser “phase stabilization” technology allowed Australian researchers to correct for atmospheric turbulence in three dimensions. This was largely the effort of Ph.D. student Benjamin Dix-Matthews, who explains that the technology allowed them “to send highly-stable laser signals through the atmosphere while retaining the quality of the original signal. … It’s as if the moving atmosphere has been removed and doesn’t exist.”
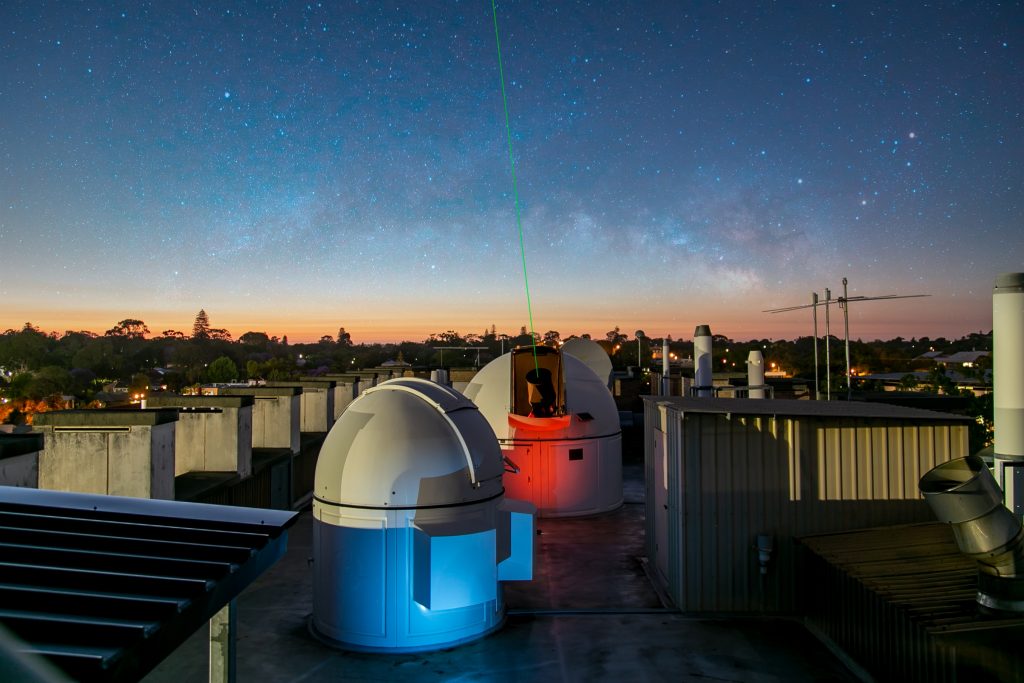
This kind of stabilization, once it can be deployed on a regular basis, will allow new kinds of physics experiments to be done. This story caught my attention because it will allow us to actually measure how the ticking of time varies from the surface of our Earth to different orbits due to the effects of Einstein’s relativity.
Beyond enabling new science, these lasers will also be useful for high-speed data transmission. While today’s radio waves travel at the same speed of light as these lasers, the lasers can support higher data densities, allowing more information to flow per minute and microsecond. According to co-author Sascha Schchediwy: The next generation of big data-gathering satellites would be able to get critical information to the ground faster.
This new technology was developed to allow the upcoming Square Kilometer Array to synchronize signals between dishes, but it once again has shown us that the technology we need to do astronomy can lead to improvements in our fundamental understanding of the universe and breakthroughs in how we communicate around the world. As a reminder, it was researchers in Australia who figured out how to invent wifi while working on radio astronomy research.
Using lasers and light to communicate has long been the stuff of science fiction, and our final story definitely seems to be straight out of some hopefully not too dystopian sci-fi future.
Researchers from Nanjing University in China have used drones to transmit photons — light particles — across a kilometer of air and have found they can keep the particles quantum-entangled through this journey.
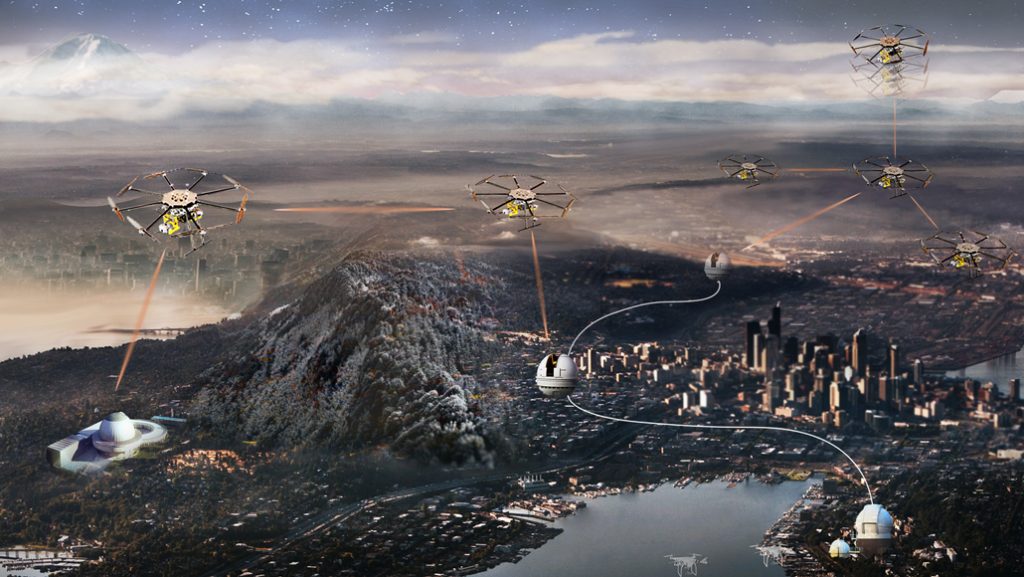
Let me try and break this down. If you generate a pair of particles together, they have to balance out a lot of different characteristics like a matched yin and yang of particle physics. If one of the balanced characteristics in one of the entangled particles changes, somehow the other particle will instantly change to keep things in balance. How this “spooky action at a distance” works is not understood but understood or not, folks would like to take advantage of this property to allow instantaneous communications across long distances.
In this particular experiment, equipment on one drone generated two particles and transmitted one of the particles to another drone and the other particle to a ground station, which then relayed that particle to another ground station. Through all of this, the particles stayed entangled.
This work is described in the journal Physical Review Letters, and it raises the possibility of drone-enabled quantum networks becoming a real thing someday in the future. Considering how loud drones can be and how much of a hazard they can be for airplanes, I’m not sure if this is something I want to see become our reality, but if Amazon can someday deliver packages with drones, we can also use them to set up particle relay stations.
Whatever happens, the future will be weird and awesome and require a lot of quantum mechanics.
This has been the Daily Space.
Learn More
Lots of water in the world’s most explosive volcano
- Washington University in St. Louis press release
- “Evidence for superhydrous primitive arc magmas from mafic enclaves at Shiveluch volcano, Kamchatka,” Andrea E. Goltz et al., 2020 November 18, Contributions to Mineralogy and Petrology
TOI-178 With Six Planets in Orbital Resonance Challenges Formation Theories
- ESO press release
- “Six transiting planets and a chain of Laplace resonances in TOI-178,” A. Leleu et al., to be published in Astronomy & Astrophysics
First Cloudless Hot Jupiter Discovered
- Center for Astrophysics | Harvard & Smithsonian press release
- “Evidence of a Clear Atmosphere for WASP-62b: The Only Known Transiting Gas Giant in the JWST Continuous Viewing Zone,” Munazza K. Alam et al., 2021 January 11, The Astrophysical Journal Letters
TRAPPIST-1 Exoplanets All Have Very Similar Compositions
- University of Liège press release
- NASA Jet Propulsion Laboratory press release
- “Refining the Transit-timing and Photometric Analysis of TRAPPIST-1: Masses, Radii, Densities, Dynamics, and Ephemerides,” Eric Agol et al., 2021 January 22, The Planetary Science Journal
Tidal Dwarf Galaxy Provides Evidence of Star Formation
- University of Bath press release
- “ALMA resolves giant molecular clouds in a tidal dwarf galaxy,” M. Querejeta et al., 2021 January 22, Astronomy & Astrophysics
Galaxy Collisions Can Starve Massive Black Holes
- The University of Tokyo press release
- “Destruction of the central black hole gas reservoir through head-on galaxy collisions,” Yohei Miki, Masao Mori, and Toshihiro Kawaguchi, 2021 January 25, Nature Astronomy
World Record Laser Link Could Help Prove Einstein Was Right
- ICRAR press release
- “Point-to-point stabilized optical frequency transfer with active optics,” Benjamin P. Dix-Matthews et al., 2021 January 22, Nature Communications
Quantum Internet Possible With Drones
- Science News article
- “Optical-Relayed Entanglement Distribution Using Drones as Mobile Nodes,” Hua-Ying Lui et al., 2021 January 15, Physical Review Letters
Credits
Written by Pamela Gay and Beth Johnson
Hosted by Pamela Gay and Beth Johnson
Audio and Video Editing by Ally Pelphrey
Content Editing by Beth Johnson
Intro and Outro music by Kevin MacLeod, https://incompetech.com/music/