Join us as we take a deep dive into the history of atmospheric methane on Mars and Titan, how that methane could be a sign of life, and what methane means for future missions and science. Plus, a planetary nebula, a supernova, ancient helium, and a couple of rockets.
Podcast
Show Notes
How a planetary nebula gets its shape
- NRAO press release
- “The Rapidly Evolving AGB Star, V Hya: ALMA finds a Multi-Ring Circus with High-Velocity Outflows,” R. Sahai et al., to be published in The Astrophysical Journal (preprint on arxiv.org)
Supernova remnant expands unevenly
- NOVA press release
- “The forward and reverse shock dynamics of Cassiopeia A,” Jacco Vink, Daniel J. Patnaude, and Daniel Castro, to be published in The Astrophysical Journal (preprint on arxiv.org)
Stunning galaxy teaches us we’re small and full of clouds
- NASA image release
Gas from Big Bang found inside Earth
- AGU press release
- “Primordial Helium-3 Exchange Between Earth’s Core and Mantle,” Peter L. Olson and Zachary D. Sharp, 2022 March 28, Geochemistry, Geophysics, Geosystems
NASA rockets to explore aurora
- NASA Goddard press release
- University of Alaska Fairbanks press release
China launches a new rocket
- CASC press release (Chinese)
Of Titan and methane
- UCSC press release
- “The case and context for atmospheric methane as an exoplanet biosignature,” Maggie A. Thompson et al., 2022 March 30, PNAS
- “A post-Cassini view of Titan’s methane-based hydrologic cycle,” Alexander G. Hayes, Ralph D. Lorenz, and Jonathan I. Lunine, 2018 April 30, Nature Geoscience
- The Mystery of Methane on Mars and Titan (Scientific American)
- A Mission to Explore the Methane Lakes on Titan (Universe Today)
- NASA Goddard press release
- Cassini Explores a Methane Sea on Titan (NASA)
- Curiosity Finds Methane, Organic Molecules on Mars (Sci-News)
- Dragonfly, NASA’s mission to Saturn’s moon Titan (The Planetary Society)
Transcript
Today, in the moments leading right into recording, we found out one of the more common unannounced surprises had occurred.
China launched a rocket. A really awesome rocket. That we’ll talk about ever so briefly.
Because we already had amazing news on so many things.
Planetary nebulae, supernovae, pretty galaxies, pretty gases.
And methane – methane as a tracer of life… maybe.
And rockets launching tracers… of barium. Not for life, but for magnetospheric research to help satellites.
So, we have all that – and a surprise Chinese rocket – right here on the Daily Space.
I am your host Dr. Pamela Gay.
And I am your host Erik Madaus.
And we’re here to put science in your brain.
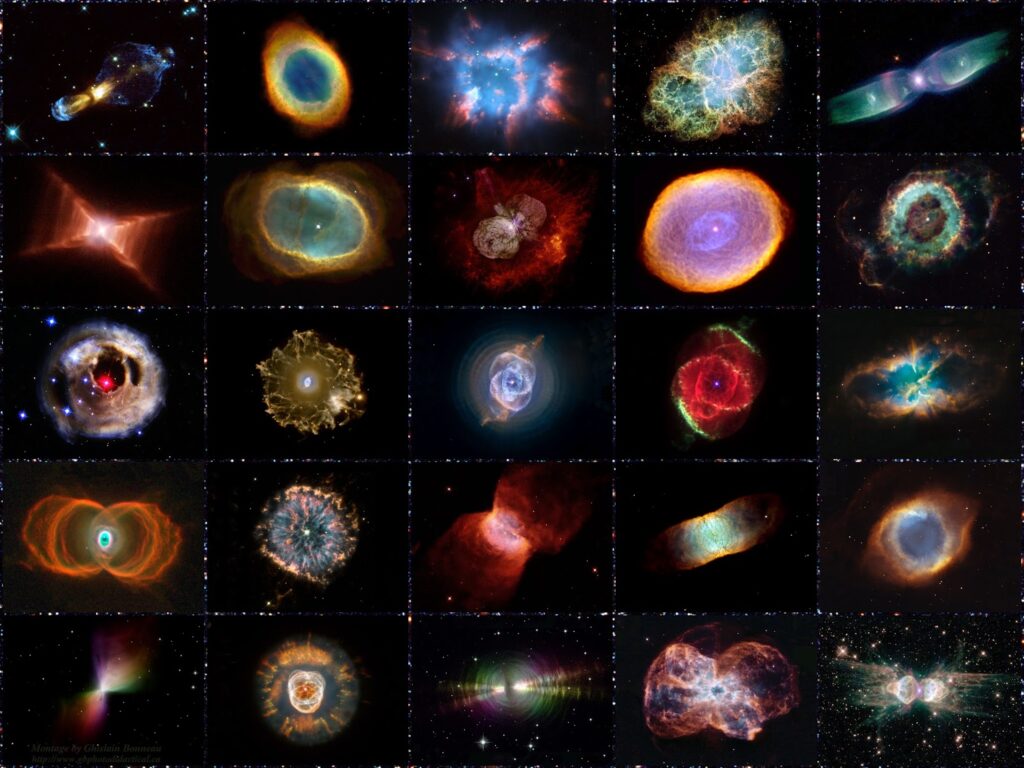
One of the scientific ideas we find ourselves stating pretty regularly around here is that stars with a mass not too different from our Sun will eventually exhale their atmospheres as planetary nebulae, forming a fabulous structure that is likely spherical or hourglass-shaped. The evolutionary phase where this mass loss really kicks in is called the Asymptotic Giant Branch or AGB, and these elder red giants are massive, low-density, mass-losing beasts.
The shapes of planetary nebulae come from the material being blown off in spherical winds from the entire star and, in some cases, being pinched off where a disk of planets or other materials prevents material from expanding. This simple model explains both the basic spherical or hourglass shapes and allows for the variety of structures we see to be the result of the material coming off in puffs and being shaped by binary companions and planets that have evolving orbits.
But as much as we like our nice simple model, sometimes it just doesn’t hold up, and new observations of one star, V Hydrae, don’t want to fit our model at all. As researcher Raghvendra Sahai puts it: Our study dramatically confirms that the traditional model of how AGB stars die—through the mass ejection of fuel via a slow, relatively steady, spherical wind over 100,000 years or more—is at best, incomplete, or at worst, incorrect.
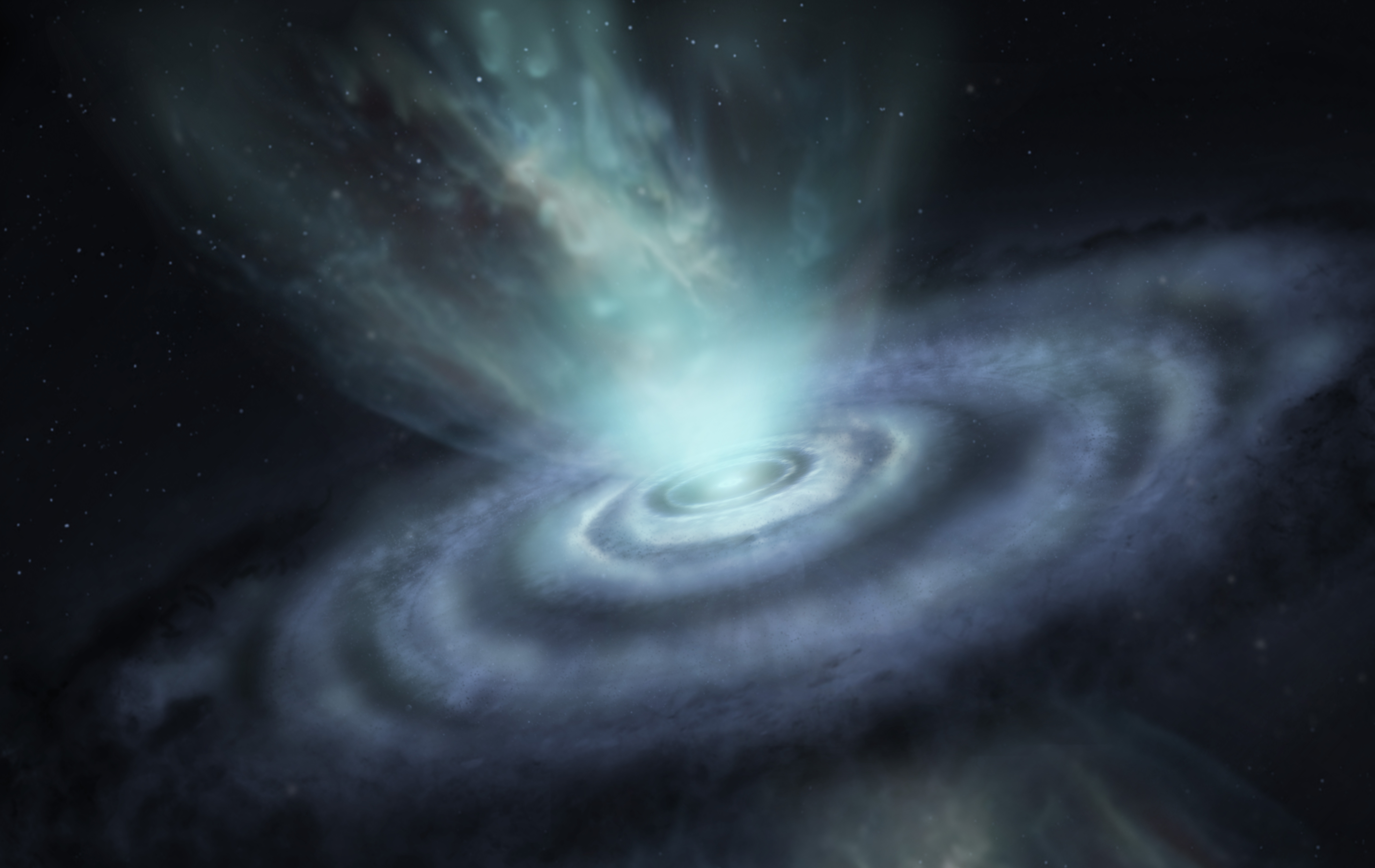
These comments come from looking at V Hydrae with the Atacama Large Millimeter/submillimeter Array (ALMA) and discovering this particular AGB star is surrounded by an expanding disk of six slowly expanding rings as well as two hourglass-shaped structures. The hourglass is fine – normal even – but that disk implies some interesting gravitational pulls, and Sahai goes on to say: It is very likely that a close stellar or substellar companion plays a significant role in their deaths, and understanding the physics of binary interactions is both important across astrophysics and one of its greatest challenges. In the case of V Hya, the combination of a nearby and a hypothetical distant companion star is responsible, at least to some degree, for the presence of its six rings, and the high-speed outflows that are causing the star’s miraculous death.
While it was previously known that V Hydrae has fast outflows, the structure of this system, located 1,300 light-years away, wasn’t well understood until ALMA took a look. The NSF program officer for ALMA, Joe Pesce, states: The capabilities and resolution of ALMA are finally allowing us to witness these events with the extraordinary detail necessary to provide some answers and enhance our understanding of an event that happens to most of the stars in the Universe.
In this case, the higher resolution images are showing us that what we thought was true but simplified is even more simplified than we thought, and we’re going to need to add a lot more detail before we can really say we know what is happening when stars die.
And that complexity needs to include parameters for not just disks of planets but also companion stars of all possible configurations. Collaborator Mark Morris explains: We suspect that it might be related to the presence of orbiting companion stars, but it is difficult to explain that given the few-hundred-year interval between ring ejections. This star is providing a new and fascinating wrinkle to our understanding of how stars end their lives.
This work is published in The Astrophysical Journal with Sahai as the lead author.
Remarkably, the complexity we’re seeing now is only the tip of the iceberg. We still need more data in more colors of light, and as Sahai reminds us: Each time we observe V Hya with new observational capabilities, it becomes more and more like a circus, characterized by an even bigger variety of impressive feats. V Hydrae has impressed us with its multiple rings and acts, and because our own Sun may one day experience a similar fate, it has us at rapt attention.
For now, we wait, and when those new observations are published, we’ll bring you the results right here on the Daily Space.
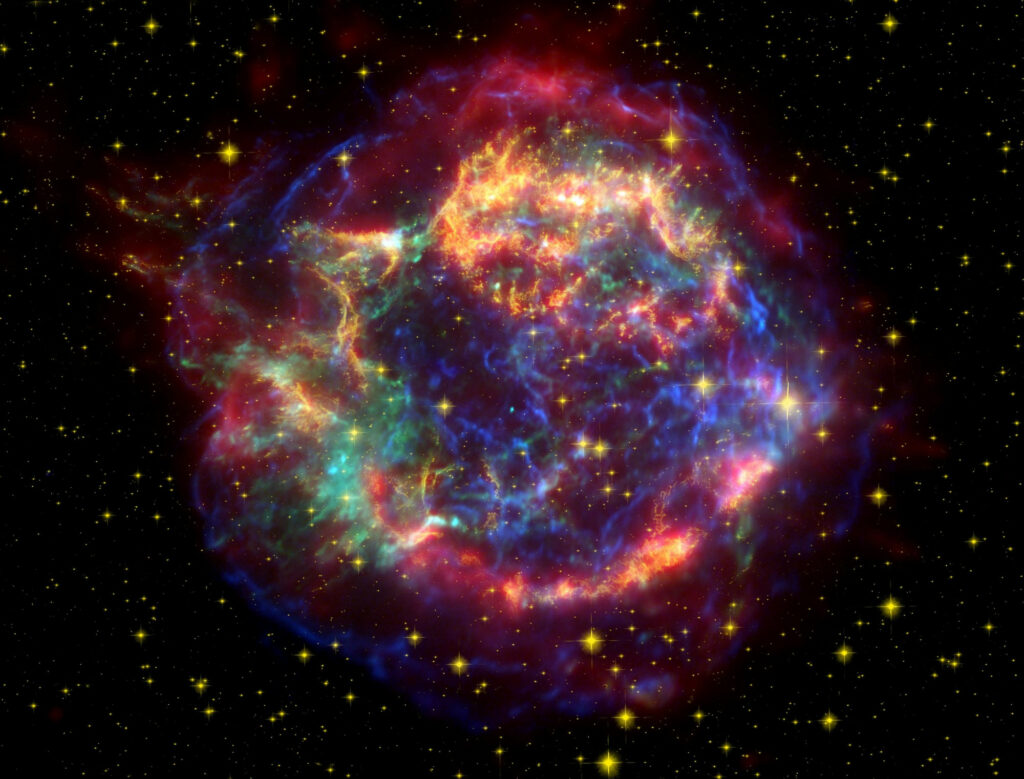
Stars are messy, especially in death. While mid-mass stars simply puff away their atmosphere, leaving the material in a gently expanding cloud of gas, the largest stars instead explode as supernovae and spew materials at high velocity in either a sphere or an hourglass shape. Initially, we think, these explosions should be symmetric, but just like with the planetary nebulae, environmental conditions can affect how the resulting nebulae are shaped.
The Cassiopeia A supernova remnant was formed in a stellar explosion vaguely around 1690. There is no definitive record of the event, although a note on August 16, 1680, by John Flamsteed may be of the initial explosion.
But we’re not worried about that exact event. What matters is that the explosion, on cosmological scales, was super recent, and we have been observing it with cameras since cameras started to be used in astronomy. With several decades of detailed images, astronomers have the ability to measure in detail how the system is expanding against the background of stars.
Or not.
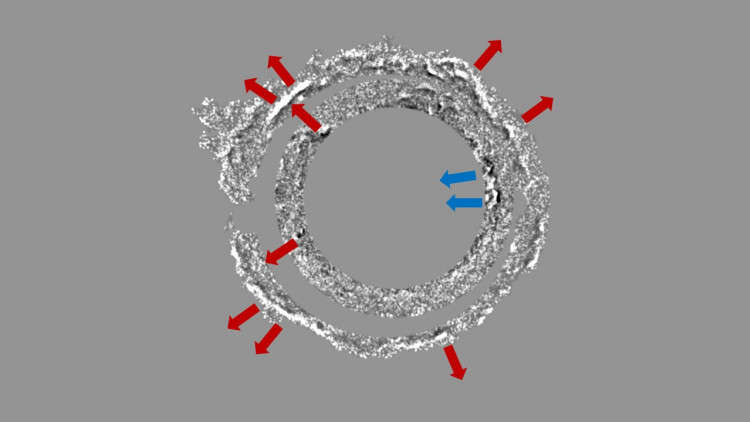
It turns out that some regions of the nebula are expanding out but a few are either stopped or actually moving inwards. In a new paper led by Jacco Vink and published in The Astrophysical Journal, researchers map out the distribution motions of knots of gas and determine, and here I quote Vink: The backward movement… can mean two things. Either there is a hole somewhere, a kind of vacuum, in the supernova material, causing the hot shell to suddenly move inwards locally. Or the nebula has collided with something.
The inward motion best matches models for a collision, which predict the kind of speed changes being seen.
Space is lumpy and bumpy and full of things that are waiting darkly to be collided with. Just as material from a firecracker can bounce off something in its surroundings, supernova remnants, we now know, can also bounce off things in their surroundings.
When we look out at space, we see myriad structures, and as we try to understand them, we keep one general principle in mind: We can’t assume we are at a special time or in a special place; what we see out there should be what exists around here.

We know we live in a spiral galaxy, and as we look out, we see plenty of sibling systems like the spiral galaxy NGC 4571 in the Virgo cluster. That particular galaxy, with its mess of arms, is structured a bit different, but its glowing clouds of star formation hint at how we may look to folks looking at us from other galaxies.
As humans, it is easy to think that we must live in a massive galaxy — a giant among giants that is forever eating dwarf galaxies for breakfast, lunch, and dinner.
While the eating dwarfs part is true, our galaxy is only kind of middling in size and is dwarfed next to the massive elliptical galaxies that are in the hearts of galaxy clusters. As we look out at sister systems like NGC 4571, we see that smallness. That other spiral with its many arms is just one of the myriad galaxies in the Virgo cluster, which itself is a part of the greater Virgo Super Cluster which is made of clusters and groups like our own Local Group.
Hubble and ALMA are currently working together to observe stunning systems in detail so we can better understand star formation, galactic structures, and quite by accident, the smallness of our place in space.
Our place is a good one, however small it may be, and up next we look at how even our tiny world holds clues to the origins of the universe.
New research quantifies the amount of a gas called helium three and proves that it comes from the Earth’s core.
Billions of years ago when the Earth was forming, it accumulated large quantities of an isotope of helium called helium three (He-3). The one place in the protosolar system where large amounts of He-3 would be is in the solar nebula, so the existence of this gas is a clue to the Earth’s formation.
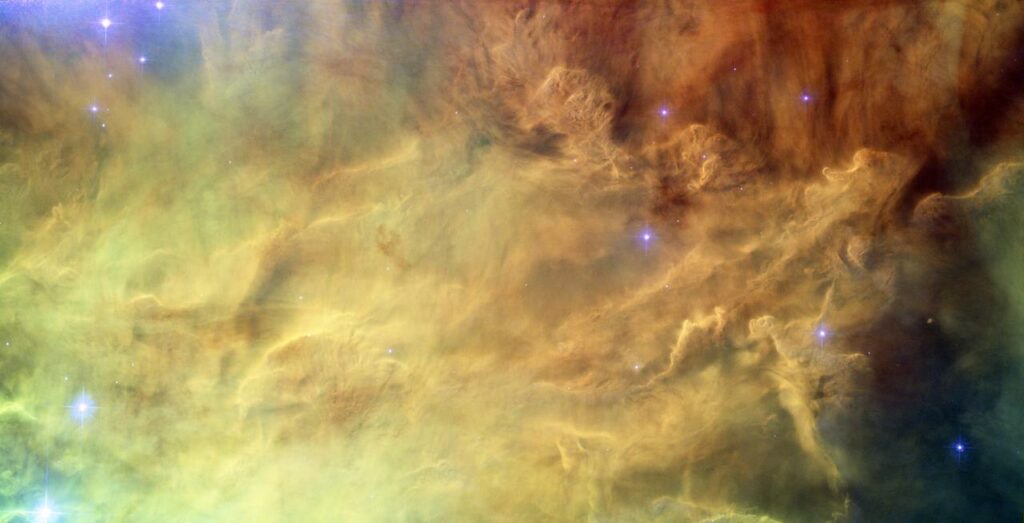
Based on the amounts detected, the helium three in Earth’s core had to come from that solar nebula at formation while the planet was still fairly molten. Later on, the Earth cooled and stopped taking in helium until the impact of a Mars-sized object melted parts of the Earth again and also formed the Moon. This caused the Earth to begin to release He-3 from the core into the mantle, a process that continues to this day. From the mantle, helium three reaches the surface through a process called deep mantle convection.
According to the researchers, two kilograms of the gas comes out of the Earth every year. Two kilograms isn’t much – about enough to fill a balloon. The models in this study, published in the journal Geochemistry, Geophysics, Geosystems, indicate that there is up to a petagram – ten to the fifteenth – grams of the gas still locked up under the surface. Lead author Peter Olson notes: It’s a wonder of nature, and a clue for the history of the Earth, that there’s still a significant amount of this isotope in the interior of the Earth.
One complication with the model is that mantle plumes don’t bring much material from the locations where helium three is concentrated deep in the mantle. These regions, called Large Low Shear Velocity Provinces (or “blobs” from a story a couple of weeks ago), are relatively stable, remaining unchanged in the mantle for hundreds of millions of years. Instead, the plumes that bring the most helium three to the surface form away from these reservoirs, closer to the core where it is hotter.
Overall, there were three things needed for this model to work – helium three dissolving into the magma ocean and separating from the core metals, then helium three depletion of the mantle, and finally, the ability of the helium three to cross the core-mantle boundary. Honestly, that’s a lot of caveats for one model to hold, but it’s still plausible, especially due to Earth getting whacked by that Mars-sized body early on in its formation.
The researchers plan further studies looking at other gasses from nebulae in the core such as Xenon 129, which if it comes from the same places as the helium three, could provide more evidence for the solar nebula theory.
We look forward to seeing just where this research leads.
And now, some rockets. Erik?
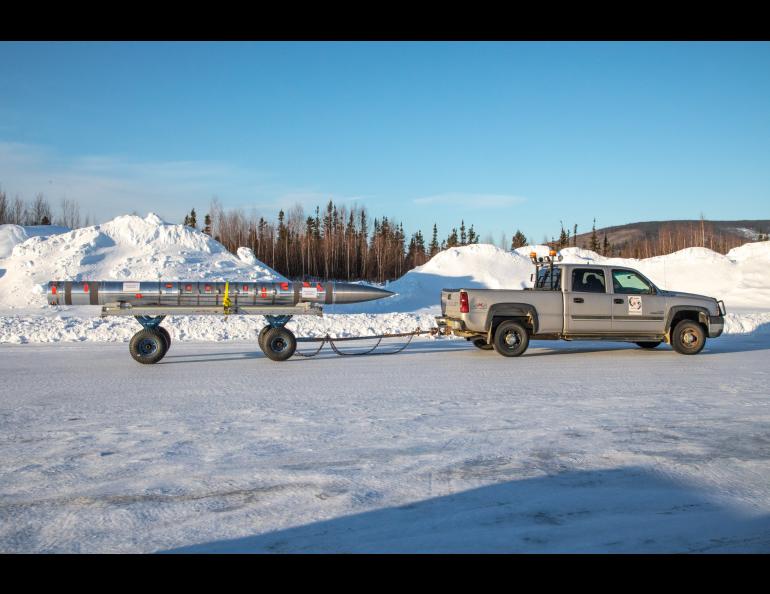
It seems this month is aurora exploration season for NASA’s fleet of sounding rockets because this coming Friday, April 1, another pair of suborbital rockets will launch to investigate something connected to aurorae.
As the charged electrons which cause aurorae streak from the Sun to Earth, they first encounter the magnetosphere and then something called the boundary layer hundreds of kilometers above the Earth’s surface. The boundary layer is where the very limits of the atmosphere interact with the Earth’s magnetosphere. This boundary layer is composed of two parts – the ionosphere and thermosphere – and the transfer of energy from the solar wind is complicated by the ionosphere being electrically charged while the thermosphere is not charged, or neutral.
The experiment called Ion-Neutral Coupling during Active Aurora (INCAA) will investigate what happens when the solar wind hits this boundary layer. It will figure out exactly what height the energy is transferred into the ionosphere and the thermosphere, the highest layer of the proper atmosphere. Aurorae can change the ionosphere locally and therefore where the energy goes, which is why this experiment needs to happen during an aurora. One known place for the energy to go is the less rarified (thicker) part of the atmosphere, interfering with orbiting satellites.
A recent example of this was the Starlink 4-7 mission in early February of this year, where a solar storm inflated the atmosphere and ultimately caused 38 of the 49 satellites to stay in safe mode and not raise their orbits, causing them to reenter the atmosphere only a few after launch.
The INCAA experiment will see two rockets launched in short succession from the Poker Flats Research Range in Alaska. The first rocket will deploy small amounts of barium to get ionized by the aurora at several altitudes up to 128 kilometers, and a second, smaller rocket will carry instruments to take data. Ground-based instruments will also be used to observe the clouds.
And now an orbital rocket launch. On March 29 at 09:50 UTC, a Long March 6A launched two satellites into orbit from the Taiyuan Satellite Launch Center in China. The Long March 6A is China’s first rocket with solid side motors, a common feature on U.S. rockets. Other Chinese rockets use liquid propellant side boosters.
Up next, we take an in-depth look at how methane may just be an indication of life.
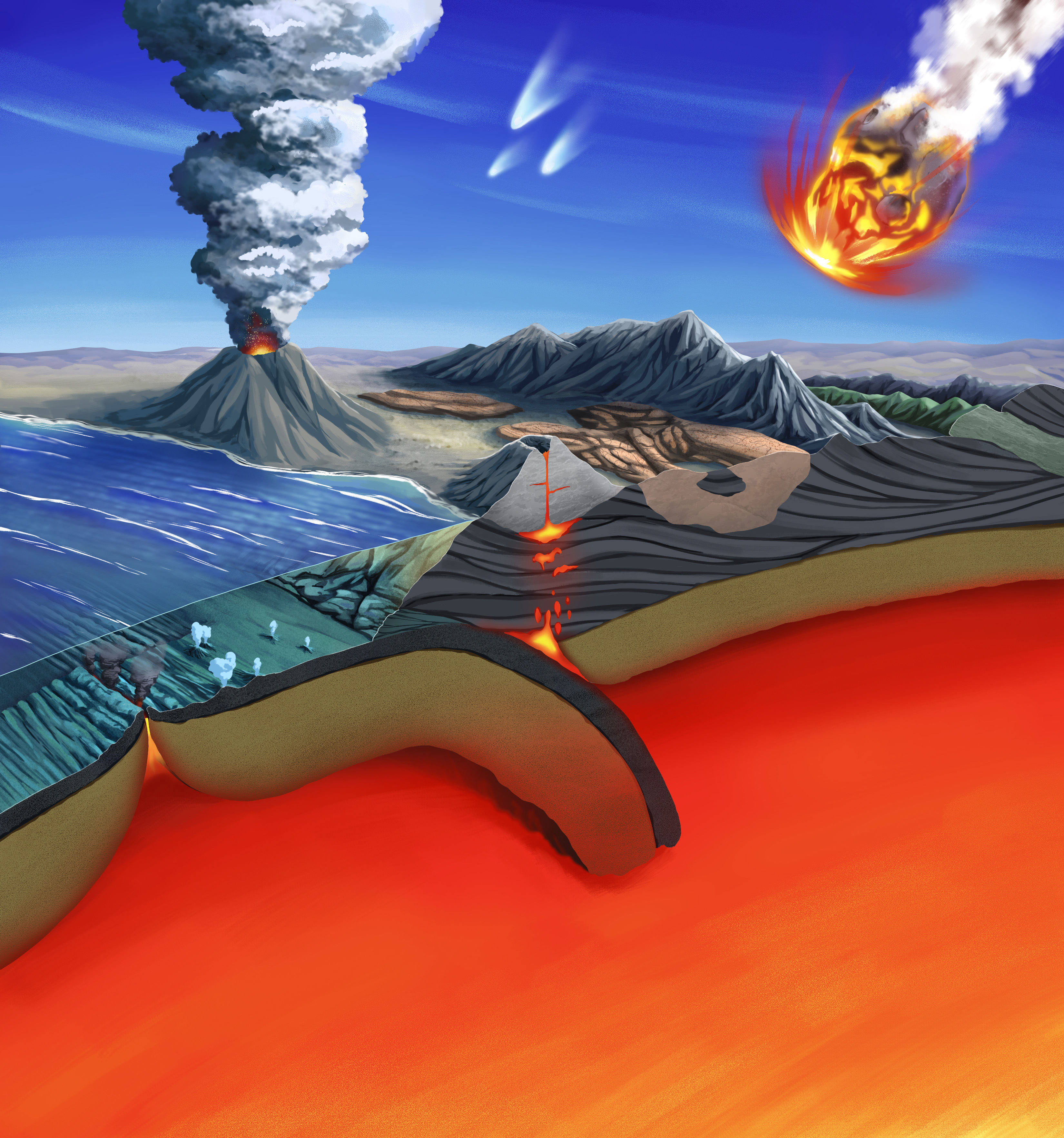
There is a place where planetary science and astrobiology start to cross over, and that is generally where we start questioning just how to go about finding life on other worlds. So far, we haven’t found anything anywhere, which is a huge disappointment to pretty much everyone. Right now, all we have are hypotheses about where life could exist, and we continue to expand on that list sort of as a thread of optimism that we’re not alone. Frankly, I’d be happy if we found some microbes somewhere that isn’t Earth, and we’ve definitely been adding to the possibilities the more extremophiles we find.
But how do we go about detecting said life if it’s there? And where should we look? Sure, we’ve found life here in sulfur pools and at the bottom of the ocean, under the ice caps, at the tops of mountains living in the snow. I mean, the planet is teeming with life. What’s next?
A new study published this week in Proceedings of the National Academy of Science focuses on one particular potential sign of life: methane. In particular, this study seeks to list out the circumstances under which biological sources of methane should be considered when it comes to a rocky world.
First off, let’s talk about the non-biological sources of methane. These include volcanoes, some underwater reactions near mid-ocean ridges and hydrothermal vents, as well as asteroid and comet impacts. Volcanoes, however, would also add other gases to the atmosphere, so if we detect carbon monoxide and methane, we could probably rule out life there. Life tends to consume carbon monoxide, so perhaps if we found methane and carbon dioxide instead, we have a sign of life.
Second, methane is incredibly unstable in the atmosphere because sunlight breaks it down. Coauthor Joshua Krissansen-Totton explains: If you detect a lot of methane on a rocky planet, you typically need a massive source to explain that. We know biological activity creates large amounts of methane on Earth and probably did on the early Earth as well because making methane is a fairly easy thing to do metabolically.
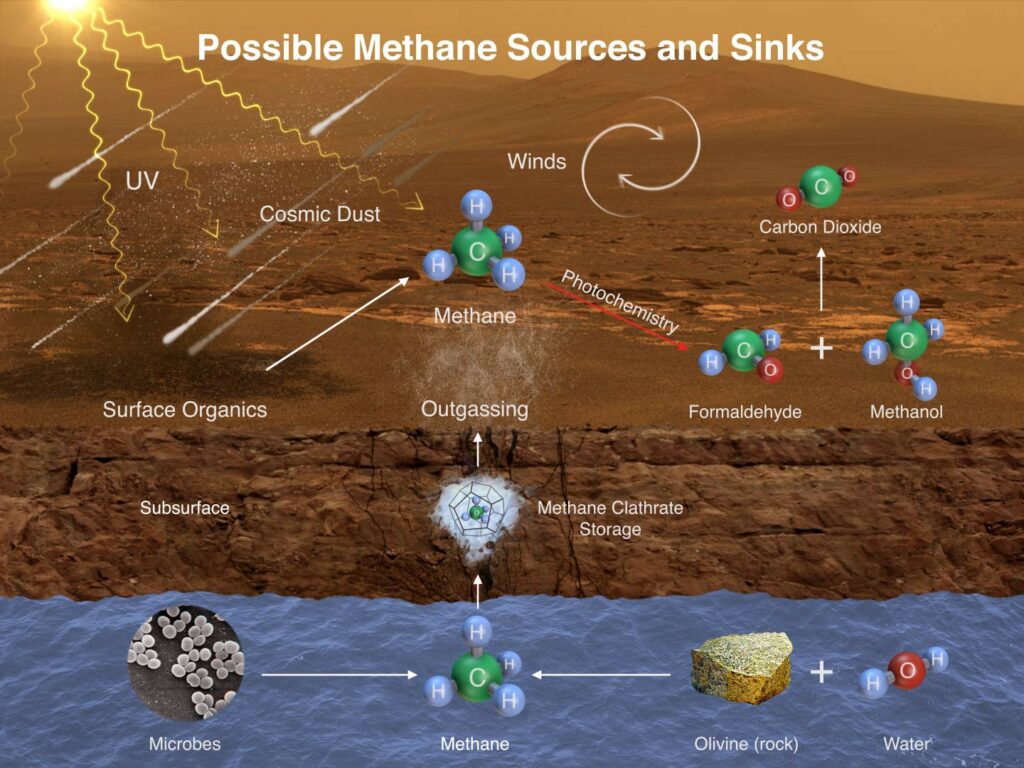
Now all of this methane speculation mostly came about because we keep detecting methane on Mars. NASA’s Curiosity rover has detected multiple surges in the amount of atmospheric methane on the red planet. ESA’s Mars Express has confirmed several of the detections as well, but no one has a solid answer for why they keep occurring. They manage to rule out reasons, such as wind erosion releasing methane from the rocks on the planet, because the amounts aren’t enough.
And there’s the Trace Gas Orbiter onboard ExoMars, which hasn’t found the same levels higher up in the atmosphere that Curiosity has detected. That discrepancy led to an entire debate about which measurements were correct, and the answer, as most things in astronomy turn out to be, was both.
Somehow, methane is building up close to the surface at night, which is when Curiosity’s sensor is used because of the power levels it requires. Meanwhile, the Trace Gas Orbiter has to be used in daylight to measure atmospheric methane, when all of that concentrated methane from near the surface gets moved up into the circulating air and diluted by the wider atmosphere.
All of this is to say that we know methane is seeping out from Gale crater and likely other places on Mars, but it’s not building up enough to be detected in the atmosphere, and we certainly don’t know for certain what the cause of the actual methane is. Is it in the rocks themselves? Unlikely in the quantities we keep seeing with Curiosity. Is there life underground creating it? Maybe?
And now we come back to the main point of today’s segment – Titan.

Saturn’s largest moon is covered in methane. Literally. There are lakes of liquid methane on the surface of Titan, and everyone is so interested in understanding these lakes that not one but two missions are in the works to explore and understand this moon.
First up, NASA’s Dragonfly drone, which is sort of like the Ingenuity helicopter on steroids, plans to explore the surface and atmosphere of Titan. The atmosphere of the tiny world is similar to that of Earth about 3.5 billion years ago when life first emerged. Dragonfly is planned for launch in 2027 and hopes to figure out what starting ingredients are necessary.
ESA’s POSEIDON mission, which is still in the proposal stage, will have two separate vehicles – an orbiter and a lander. The orbiter will collect all that wonderful atmospheric data we need and the lander would go find a lake and collect data on the actual liquid methane.
All of this is in an effort to answer the question: Can we reliably use methane as a signature of life on other worlds? After all, more than 70% of the methane in our own atmosphere here on Earth is biologically created. It’s not impossible for the same to be true on Mars and Titan, just in smaller quantities. But before we can state that we have found zebras, we have to check for horses. And that’s why missions to these two worlds, especially ones looking for direct signs of biological activity, are incredibly important.
The broader implication here is that, once we know for certain that methane on either world is biologically produced, we can start to put together combinations of atmospheric makeup that we can then look for with a more sensitive telescope, like JWST. That means cataloging exoplanet atmospheres and maybe, possibly, finally… finding life on even more distant worlds.
This has been the Daily Space.
You can find more information on all our stories, including images, at DailySpace.org. As always, we’re here thanks to the donations of people like you. If you like our content, please consider joining our Patreon at Patreon.com/CosmoQuestX.
Credits
Written by Pamela Gay, Beth Johnson, Erik Madaus, and Gordon Dewis
Hosted by Pamela Gay, Beth Johnson, and Erik Madaus
Audio and Video Editing by Ally Pelphrey
Content Editing by Beth Johnson
Intro and Outro music by Kevin MacLeod, https://incompetech.com/music/