Do you want lasers? I want lasers! And today’s show features lots of lasers. We also have more questions than answers about Mars’ methane, misbehaving stars, and new research on how we would look for the early signs of life on other worlds. Plus, a study on how spaceflight impacts the human brain.
Podcast
Show Notes
Researchers get into the dense parts of geoscience
- How to look thousands of kilometers deep into the Earth? (EurekAlert)
- “Electronic, Structural, and Mechanical Properties of SiO2 Glass at High Pressure Inferred from its Refractive Index,” Sergey S. Lobanov et al., 2022 February 17, Physical Review Letters
Update on Mars methane
- The Mystery of Methane on Mars Thickens (Eos)
- “Mars Methane Sources in Northwestern Gale Crater Inferred From Back Trajectory Modeling,” Y. Luo et al., 2021 November 4, Earth and Space Science
- “Constraints on Emission Source Locations of Methane Detected by Mars Science Laboratory,” D. Viúdez-Moreiras, M. I. Richardson, and C. E. Newman, 2021 December 7, JGR Planets
Sun fires off a photogenic outburst
- ESA press release
Is there Sun-locked Life?
Re-examining where life began on Earth
Interstellar sail will need new materials
- University of Pennsylvania press release
- “Relativistic Light Sails Need to Billow,” Matthew F. Campbell et al., 2021 December 23, Nano Letters
- “Multiscale Photonic Emissivity Engineering for Relativistic Lightsail Thermal Regulation,” John Brewer et al., 2022 January 11, Nano Letters
New research on spaceflights impact on the brain
- Brains of cosmonauts get ‘rewired’ to adapt to long-term space missions, study finds (EurekAlert)
- “Brain Connectometry Changes in Space Travelers After Long-Duration Spaceflight,” Andrei Doroshin et al., 2022 February 18, Frontiers in Neural Circuits
Transcript
Do you want lasers? I want lasers! And today’s show features lots of lasers.
Sadly, they are not on sharks or grad students. We have lasers in science equipment.
We also have more questions than answers about Mars’ methane, misbehaving stars, and new research on how we would look for the early signs of life on other worlds, plus a study on how spaceflight impacts the human brain.
All of this now, right here on the Daily Space.
I am your host Dr. Pamela Gay.
And we’re here to put science in your brain.
Not all research is equally exciting. Sometimes, research is just a bunch of people in a research lab trying to figure out how to most effectively crush stuff.
Seriously.
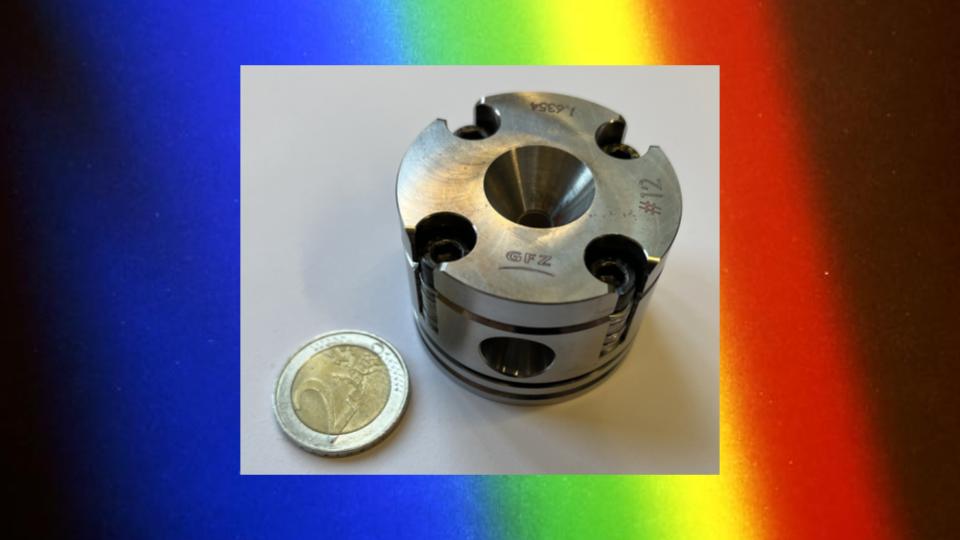
In order to understand planets effectively, we have to understand how materials behave at the extreme temperatures and pressures that exist inside of worlds like ours. Consider just the Earth’s mantle. The slow-moving ooze that carries the planet’s crust is generally more of a solid than a liquid, but if the temperature is changed just a small amount, for instance from interactions with water, the oozing material can phase change to a liquid mantle and become the stuff of volcanic eruptions.
In a perfect experiment, we’d send equipment burrowing into the Earth to record all the things that are happening. We don’t live in that reality, however, and the kinds of equipment we can build today can neither get that deep nor survive what they would encounter. Instead, researchers have to replicate the conditions found deep in the Earth inside a lab and then see how different materials act – what their density and configuration is – under those conditions.
Getting small samples to hot, dense conditions is something we know how to do, and for materials with a regular structure, we can use X-ray diffraction to see how the crystals in a material compress and measure a material’s density as the external pressure changes.
Unfortunately, not everything inside our world has a nice regular structure, and measuring the densities of more chaotic materials hasn’t been possible until now.
New research published in Physical Review Letters and led by Sergey Lobanov uses a laser to measure the volume of tiny samples, and then, as students in a freshmen physics lab would, the density can be calculated from the known mass of the sample. But unlike freshmen physics students, they didn’t just measure the outer dimensions of their sample.
Any material sliced thin enough becomes semi-transparent, and the samples in this research were just that kind of small; using multicolored lasers, they were able to measure the path of light through the inside of the material and measure its size with light passing through it. This has to do with another freshmen physics topic: refraction. Which is kind of cool and means that for the first time, we can measure the sizes of things too small to measure with anything other than light and too disorganized to measure with X-ray diffraction.
And this will eventually help us better understand the inside of our planet. As Lebonov explains: Earth was a giant ball of molten rock 4.5 billion years ago. To understand how Earth has cooled and produced a solid mantle and crust, we need to know the physical properties of molten rocks at extreme pressure. However, studying melts at high pressure is extremely challenging, and to circumvent some of these challenges geologists choose to study glasses instead of melts. Glasses are produced by quickly cooling hot but viscous melts. As a result, the structure of glasses often represents the structure of melts they were formed from. We have now shown that the evolution of the sample volume and density of any transparent glass can be accurately measured up to pressures of at least 110 GPa using optical techniques.
This just goes to show that sometimes big machines in boring labs, using freshmen physics principles, can do truly amazing things with tiny samples of our world.
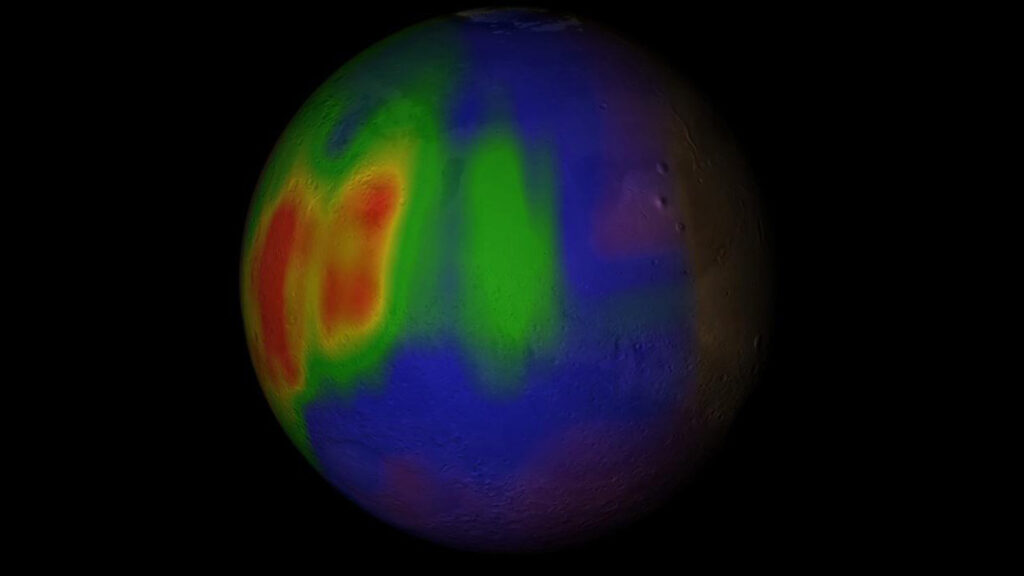
Understanding planets is harder than it seems like it should be. Us astronomers are off modeling the evolution of the universe from first principles as homework sets, while planetary researchers are struggling to make sense of basic chemistry happening right now, where we can see its effects.
To be fair, organic chemistry is a subject I dreaded so much, I convinced my advisor to let me take graduate-level stellar nucleosynthesis as a replacement for basic undergraduate organic chemistry.
One of the biggest organic chemistry mysteries of our solar system is the methane on Mars. Methane is an unstable molecule that breaks down in sunlight. When we see it, we know it has to have recently come from some source — typically a biological process or a volcanic-related process. On Mars, we see seasonal methane production using the Mars Curiosity rover, but we don’t detect it in the overall atmosphere using the Trace Gas Orbiter.
In a review of recent papers, Germán Martinez, Anni Määttänen, and David Baratoux find the data is consistent with a small methane source existing on the northwestern rim of Gale Crater — a result that seems to indicate we were either very lucky in putting Curiosity where we did and finding Mars’ one lone methane source or that Mars is particularly good at destroying methane in its atmosphere and there are other sources of methane.
Scientists don’t like the “we got lucky” solution to any problem, and the idea that Mars has one and only one methane source is weird. At the same time, everything else we know about chemistry on Mars fits our understanding of chemistry, and it is also uncomfortable to think methane alone behaves super weirdly.
Bottom line: in looking through the research, they find the Curiosity measurements look solid and the lack of methane seen from orbit looks solid. And Mars is now more confusing than ever before.
Science isn’t always comforting, folks. The “I have no idea” issues are many, and sometimes the problems are just weird. Our solar system just isn’t the nice, easy-to-understand, life-supporting place we might wish. Even our Sun likes to lash out occasionally.
In breaking news, the Solar Orbiter has observed the Sun undergoing a magnificent eruption. This event is pointed away from the Earth and poses no risk to us. This particular event is also scientifically just right in size to allow some cool new research to be done. Most events like this are either so large that we lose part of the event off the side of our detector or so small that the details are lost to the brightness of the Sun. According to the ESA: ...the prominence observed by Solar Orbiter is the largest ever event of its kind to be captured in a single field of view together with the solar disc, opening up new possibilities to see how events like these connect to the solar disc for the first time. At the same time, SOHO can provide complementary views to even larger distances.
The Sun is currently coming out of a quiet period, and in the next few years, we should be treated to more solar outbursts of various kinds. Let’s just hope all of them pose nicely for the cameras while pointing their high-energy particles away from the Earth.
From planets local to Earth to planets far away, let’s jump out of our solar system and consider how life may exist out there among the stars.
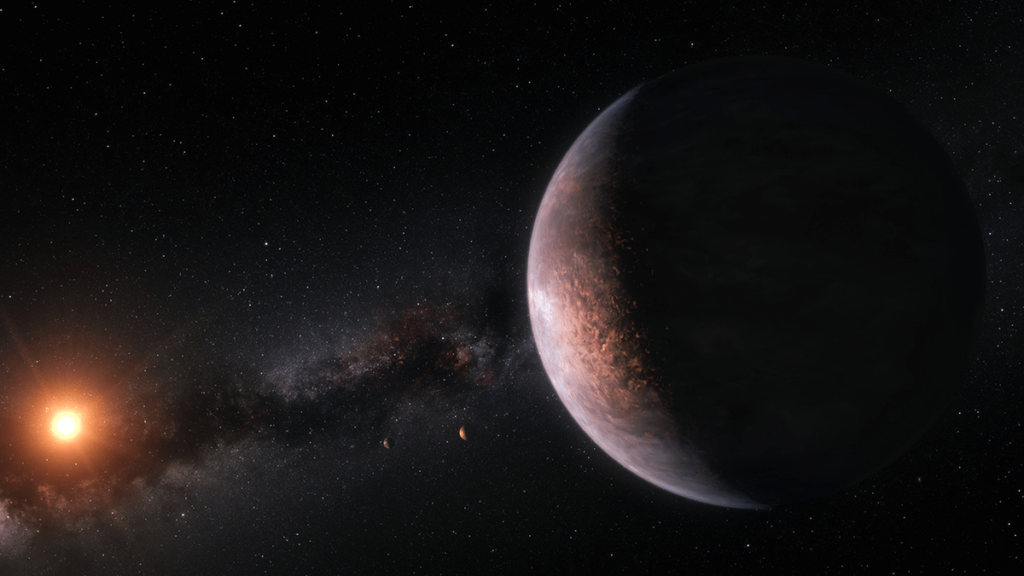
We are really lucky with our Sun. At this point, it’s generally not too active, and it’s evolving at a nice pace to give us humans time to adapt to how it is changing and how it will change our environment someday.
Other worlds have very different relationships with their star, relationships that are more akin to what the Apollo astronauts experienced as they looked at the Earth. From their vantage on a tidally locked Moon, they always saw the Earth in the sky. It appeared to rotate, to slowly change phases, but its position was constant. Here on Earth, we always see the exact same face of the Moon, and, weirdly, that means the Earth is pretty much stationary in the lunar sky.
We ended up in this configuration because the Moon is close enough to Earth that our planet’s gravity was able to slow the Moon’s past rotation with torque. A mountain would try and swing past, adding its bulk to the Moon, and gravity would say “no, mine” and pull on that extra mass, slowing the world’s rotation.
What our Earth does to the Moon, other stars are able to do to their close-in planets. This can lead to weird situations where small stars with large planets can end up with tidally locked planets sitting in their habitable zones, one side always facing the sun and one side always facing away. This means a life form on these worlds would have to travel around the globe to experience a sunrise, sunset, and all the parts of a day in between.
Which is weird to imagine. And wreaks havoc on the weather.
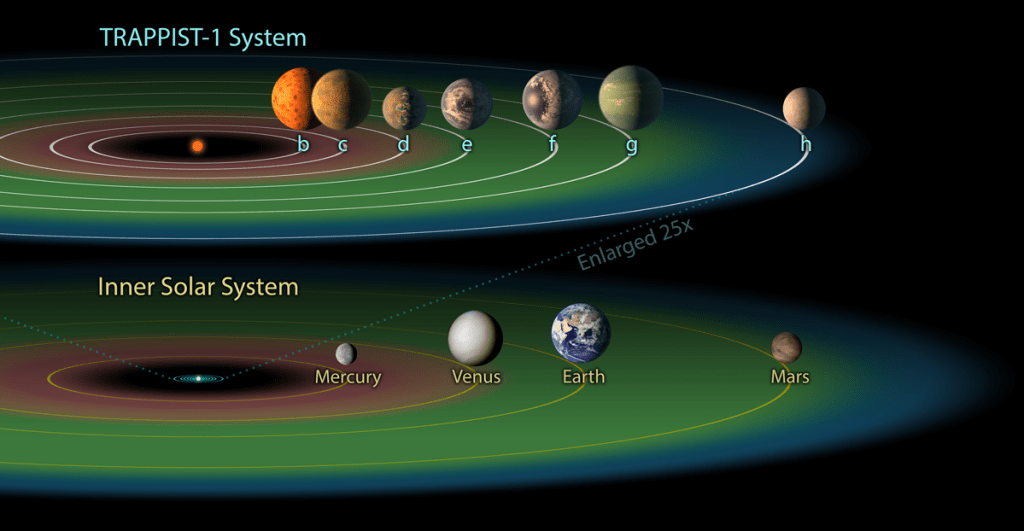
The question has become “Can these otherwise good-for-life worlds support life or do the winds and storms and temperatures created in this weird situation make life as we know it impossible?” An article in EOS reviews the literature on this matter and finds that life is possible, but we are likely looking at extreme life – life that in one instance is designed for the high temps of ever-lasting noon or the gale winds of a forever-setting sun.
This constancy actually may cause some of its own difficulties. Here on Earth, life often cycles with our seasons, but a tidally locked world will have none of that. The amount of light a place gets is just the amount of light it gets… always. We know there is subsurface and deep-sea life here on Earth that experiences this kind of constancy, but even scientists struggle to imagine how you grow a complete civilization from a place where each places climate is distinct, and spreading around the world to fill a niche in the ecosystem just isn’t possible.
The TRAPPIST-1 System is the nearest system to have a planet in the habitable zone that is tidally locked, and as we develop bigger and bigger telescopes, I look forward to new data that will allow us to better imagine this truly bizarre – to humans – kind of a situation.
Trying to understand where life might evolve in other solar systems sometimes feels like we’re jumping ahead. We still don’t know what worlds in our solar system do, don’t, and previously had life. This won’t stop us from imaging other solar systems, but it does put a bit of extra push into the work of folks trying to understand just where life on Earth originated and when it originated.
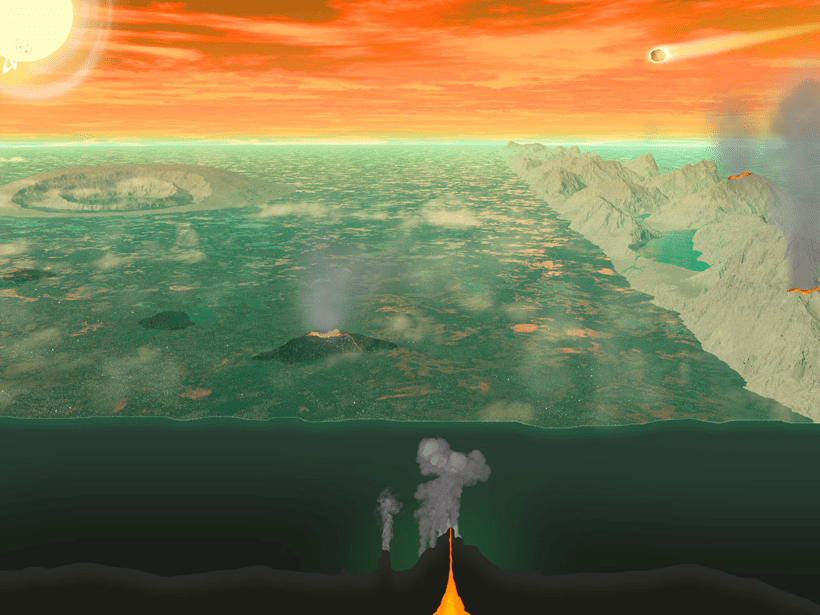
Our planet’s oldest fossils date back to 3.5 billion years ago, and finding older rock is challenging, so this isn’t as much a “life wasn’t here before that” as “our world didn’t leave us a lot of rock we can access from before that”. There are some indications of biological activity just over four billion years ago, but that’s all we’ve got.
And our ability to better understand how planets form and evolve is starting to inform how we look for life. New interdisciplinary work brings together geologists and prebiotic chemists to work together on understanding all the various possible ways life could emerge. Under consideration are environments like the interface between rock and water and factored in are everything from the ultraviolet radiation of our young Sun and the delivery of organic molecules on impacting meteors and comets.
Researchers are taking into consideration how the materials released from the planet’s interior in volcanoes can rain down and interact with water in hot springs and the general water cycle. And researchers are imagining how life may not have started under the Sun but may instead have originated in the bottom of the ocean.
This is all to say that we don’t know how life came to exist; we just know it did, and through interdisciplinary collaborations, we’re constantly defining more places that it could have come into existence. And for now, we can be excited at the possibilities of life emerging in more than one of these places and continue to hope that it emerged on more than one world not just in our galaxy but maybe even in our solar system.
And while we figure this out, we’re going to keep dreaming of visiting other worlds.
Next up, Erik Madaus brings us news on the kinds of space hardware that just might be used to get to the nearest alien worlds and the effects space flight has on our human bodies.
The Breakthrough Starshot project aims to send a tiny spacecraft to Alpha Centauri, the closest star to Earth, in far less than a human lifetime, only twenty years. Instead of chemical propulsion using hydrocarbon or noble gas propellant, the project aims to use a series of lasers to push on a large sail.
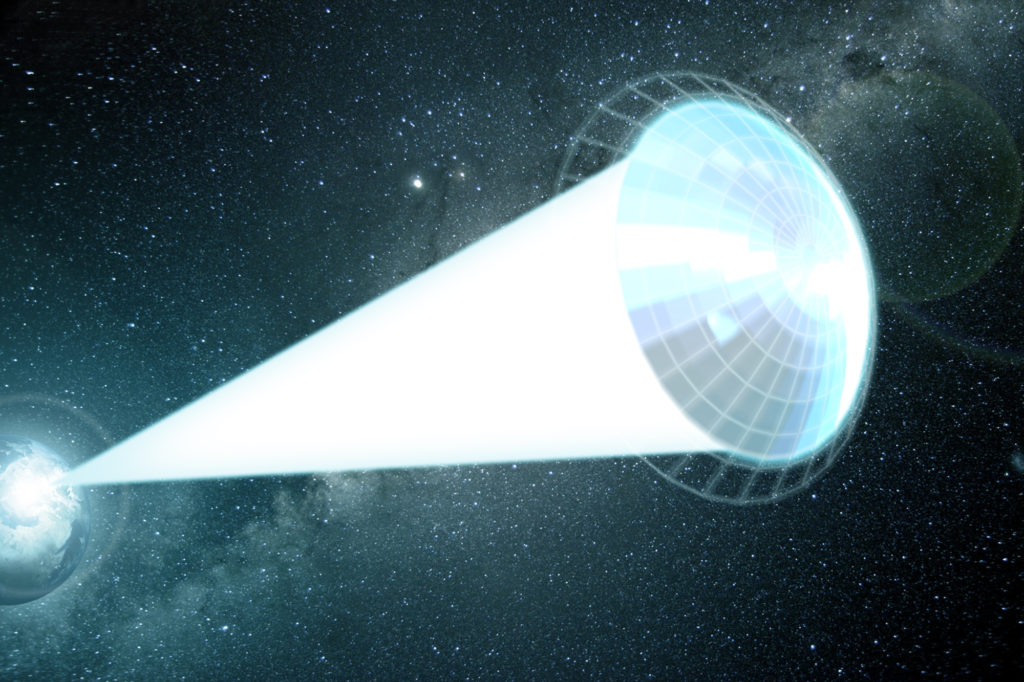
Sails have flown in space before, but they accelerated on solar pressure alone. That’s not fast enough to get to another star in twenty years. Current designs for sails cannot handle the thermal and acceleration loads of being pushed by a laser, so a team in the University of Pennsylvania Department of Mechanical Engineering and Applied Mechanics and the Department of Materials Science and Engineering at UCLA have done some work on what properties the sail needs to have.
The team published two papers in the journal Nano Letters.
One of the key insights the authors present in their first paper is the structure of the sail. It cannot be rigid, as the pressure of the lasers would rip it. Instead, it needs to be curved to withstand the thousand gee force of the lasers. The authors note that other pressurized containers, such as rocket fuel tanks, also need to be cylindrical to withstand the forces on them.
The other paper the team prepared on the dynamics of the proposed laser sail covers how the sail could dissipate all the heat generated by the lasers. As the authors say: …if the sails absorb even a tiny fraction of the incident laser light, they’ll heat up to very high temperatures.
The key is a pattern woven into the material, a combination of holes and swatches in a grid. The holes and swatches combine to match the light and thermal emission, allowing the sail to be pushed by even more powerful lasers than previously planned or permit a shorter laser burst that can be more accurately controlled.
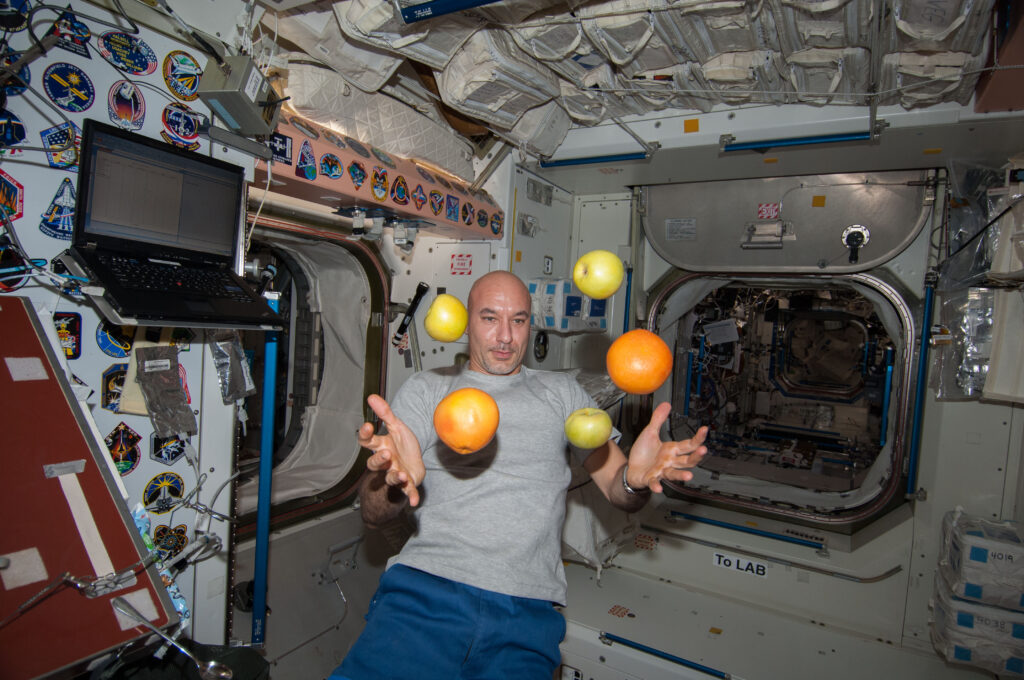
Before we can leave our solar system as humans to join that sail, we need to understand how space affects our bodies.
Science has investigated lots of the changes in the human body in reaction to spaceflight except how the brain changes, until now. A new study in Frontiers in Neural Circuitsshows structural changes in the brain as a result of spaceflight.
First, some background. Gray matter is the thinking part of the brain, and white matter allows different gray matter parts to communicate. A team of ESA and Roscosmos researchers studied the brains of twelve cosmonauts before and after spaceflights using a technique called fiber tractography using a special MRI machine. This method is essentially a “wiring scheme of the brain” according to Floris Wuyts, lead researcher of the study. The scans were conducted before, right after, and seven months after the spaceflights.
The study found that the brain can learn how to adapt to spaceflight, called neuroplasticity — specifically, the connections between different areas that controlled motion. This makes sense because moving around in microgravity is much different compared to moving around in gravity. These pathways were still present seven months after returning to Earth.
The authors also thought that the corpus callosum, the region that connects the two hemispheres of the brain, was structurally changed. It turns out that the changes were physical, brought on by changes from microgravity in the ventricles, fluid-filled regions in the brain next to the corpus callosum. Microgravity makes these regions swell up, which can increase headaches in astronauts.
The authors conclude that additional research is needed in other areas of how the brain is impacted by spaceflight and how to counteract potential damage. The studied cosmonauts were only in space for an average of 172 days, which isn’t long considering the months or years future space missions to other planets may last. This research and countermeasures exist for physical impacts to the body but not yet to the brain.
This has been the Daily Space.
You can find more information on all our stories, including images, at DailySpace.org. As always, we’re here thanks to the donations of people like you. If you like our content, please consider joining our Patreon at Patreon.com/CosmoQuestX.
Credits
Written by Pamela Gay and Erik Madaus
Hosted by Pamela Gay and Erik Madaus
Audio and Video Editing by Ally Pelphrey
Content Editing by Beth Johnson
Intro and Outro music by Kevin MacLeod, https://incompetech.com/music/