Media
Transcript
The role of luck in science is something I sometimes feel we just don’t acknowledge enough. To do amazing things, you don’t just have to be one of the best in the world in terms of intellect and research skills, you also have to sometimes get lucky that the kind of object you’re interested in goes off at the right time and in the right place for you to get the data you need.
On Thanksgiving Eve in 2018, the Swift observatory caught a short gamma-ray burst (GRB) – the kind of high energy flash that occurs when two neutron stars merge. Only a handful of these occur each year, and each is thought to be accompanied by a flash of optical light that lasts for tens of minutes to a few hours. Seeing these flashes is necessary to confirm the gamma-ray burst’s location in the universe, but these often distant phenomena are super hard to observe.
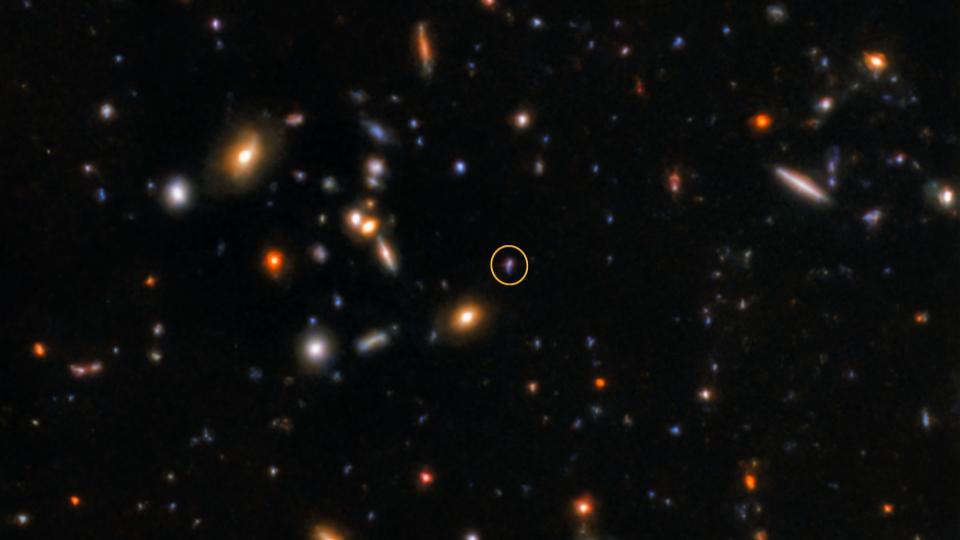
To catch these events, the bursts, first of all, need to appear in the sky over the part of the planet that is dark, and then a telescope needs to be found that can go looking for whatever may or may not be visible. Telescope time is pre-allocated to different researchers who are generally doing something other than looking for gamma-ray burst afterglows. As someone who’s gotten the pre-dawn call to “please go chase a GRB”, I can tell you that the process generally involves a fair amount of cursing, stumbling out to the dome to point the telescope at something in a radically different part of the sky, watching the telescope slowly slew, stumbling back into the control room, and then taking images based on a wild guess of what exposure times should be used.
My personal attempt to do follow-up for some researchers didn’t uncover anything, but Northwestern University’s Wen-fai Fong had a very different experience. Making the call to Gemini Observatory that Thanksgiving Eve, his team was able to get one of the best telescopes in the world to slew to the right place in the sky where they pinpointed the light of a short gamma-ray burst that went off order of ten billion years ago. Named SGRB 181123B, it is now the most distant short gamma-ray burst ever observed, and this identification seems to indicate that neutron stars can merge relatively quickly in the young universe.
Let’s put this in context: our universe formed 13 something billion years ago. The cosmic microwave background (CMB) was released 400,000 or so years after the universe formed. A few 100 million years later, stars were forming and galaxies were starting to take shape. Some of the largest stars forming in these early times evolved into neutron stars in a binary system, and those stars rapidly spiraled together to merge before the universe was even four billion years old. That light then traveled in all the colors of the electromagnetic spectrum to Earth, where the gamma-rays triggered a space telescope that detected the light at a time of day that made observing with the 8.1-meter Gemini telescope in Hawaii possible. Also, Fong had done all the things, made the necessary proposals, and been awarded all the needed privileges to have permission to take over usage of that particular telescope from whatever else it was doing on that particular night.
That, folks, is luck combined with all the necessary preparation.
And this is how we advance our understanding of the universe, one hard-won lucky break at a time. This work appears in a new paper in the Astrophysical Journal Letters.
As someone who lacks luck and attracts clouds, lighting, and power outages, I am happy to say there are areas of astronomy that just require a lot of patience and detail-focused work. This style of research is showcased in a paper led by Patrick Gauime and appearing in Astronomy & Astrophysics.
In this very different style of research, this team looked through data on the roughly 4500 red giant stars in the NASA Kepler data set and looked for stars that weren’t behaving in normal, boring, red giant kind of ways. In general, red giants are placid stars that slowly rotate and don’t have many or any starspots on their surface. These two factors are connected – a star’s magnetic field is driven in part by the stars rotation rate, and it is that magnetic field that generates spots. Smaller, younger stars like our Sun rotate quickly and have spots, but when they expand into red giants in later life, conservation of angular momentum will force them to rotate slower, like an ice skater extending their arms outward.
This is what usually happens at least.
But as I said, this team was looking for something unusual – they were looking for red giants that have starspots.
And they found them.
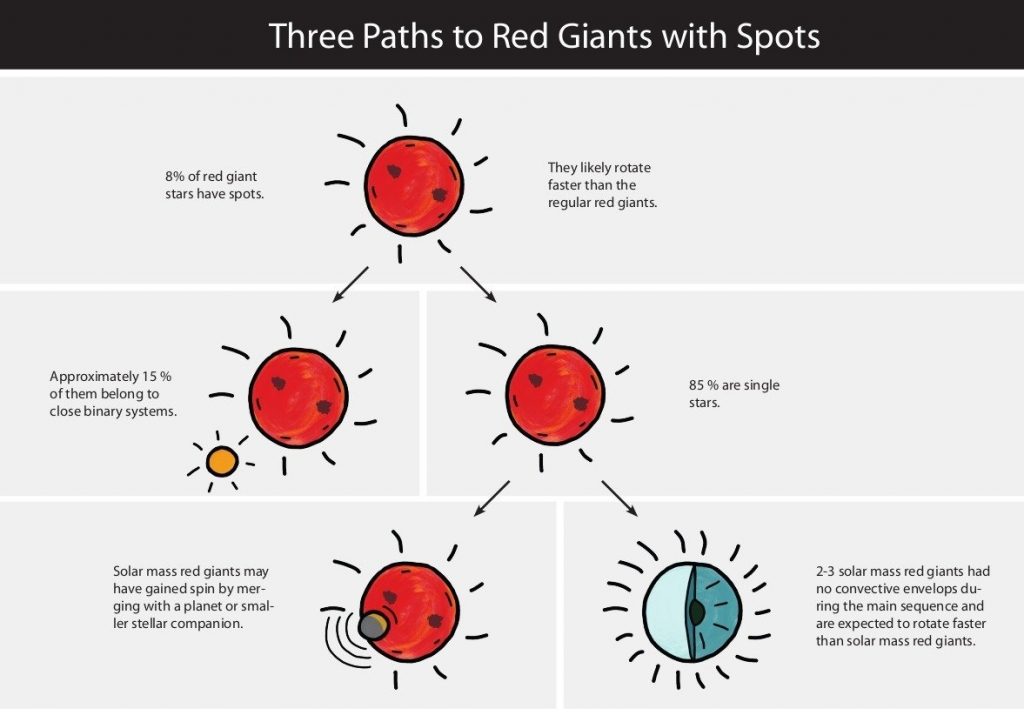
Roughly, 8% of the red giants in this survey showed the changes in the brightness characteristic of a spotty rotating star. These same stars also have short rotation periods, indicating that something spun up them and their magnetic field. It’s in trying to figure out how you spin up a giant star that things get interesting. They suggest reasons ranging from forced synchronization with another, closely neighboring star, to the swallowing of a star or planet, to a fast initial rotation speed in an early phase of development. The cool thing is, it doesn’t have to be just one reason accelerating the stars – different things could happen in different places. Now we get to see if observations can find evidence of any of these causes. If it’s found, we’ll bring it to you here on the Daily Space.
Finding weird things and then getting to explain them is why a lot of people like doing science. Large stars are one of those things that regularly demonstrate they can be more unexplainable than a giant ball of plasma seems like it should be.
Forming truly massive stars has been really hard to explain. As young stars form, they light up, and their light can push away infalling material, shutting off their growth. The bigger a star, the more brightly it will shine, and it seems like truly massive stars should shut off their own formation with their light. Since we see really massive stars, they must somehow find a way to grow. Now, in a new paper in Nature, a team of astronomers led by Xi Chen offers up a set of observations that seem to explain what is going on.
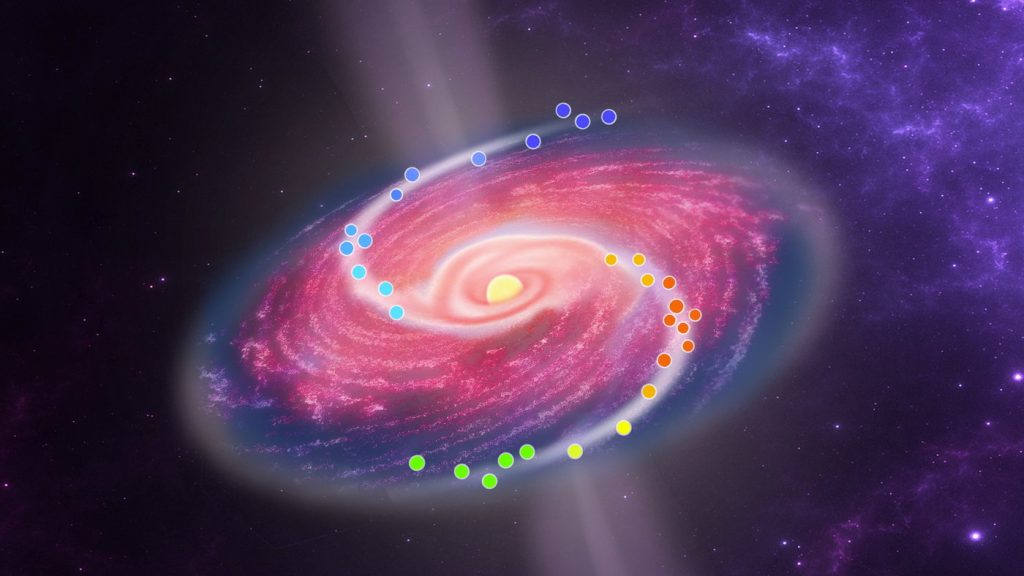
While observing the twelve solar mass proto-star G358-MM1, they were able to see light from knots in the protostellar disc that shined as masers [Ed. note: microwave amplification by the stimulated emission of radiation]. These naturally occurring microwave-colored lasers only shine when conditions – temperature, density, and the like – are exactly right, and the maser in G358-MM1 appeared to turn on and off in ways that indicated the material in this particular protostellar disc is clumped up into spiral arms, and the clumps are falling into the star in ways that cause it to periodically flare up in brightness.
According to Chen: G358-MM1 is thus the first example of a massive protostar whose sudden increase in brightness clearly coincides with the formation of a spiral, a structure that suggests an unstable, massive disk.
As they explain it, in these systems, the disk of material around this star is so massive that it becomes unstable. Its gravitational pull is such that it is pulling material from the surroundings onto itself, and as that material tries to flow toward the star, gravity from denser regions will pull more strongly, making those regions even denser, clumping the material up. The spiral structure that is seen is something that recurs throughout astronomy at different scales and wasn’t surprising. That said, seeing something you knew might be out there is always exciting because it means we actually do understand how parts of the universe we weren’t able to see work.
Our ability to understand our universe isn’t limited by human intelligence as much as it is limited by our technology. It took a more than an 8-meter telescope to observe the SGRB we started our show with. For some discoveries, we’re going to have to wait for even bigger telescopes to be built. At the same time, we also need powerful computers to model what may wait undetected for those telescopes to be built. Many of our current questions revolve around what shapes can solar systems take.
We now know that most metal-rich stars have planets. We still can’t detect small planets around most stars or distant planets at all. We only see special combinations of planetary size and distance, and everything else is outside our ability to see. We’d love to know if solar systems could have twenty planets, or four Jupiters, or a lot of wild science fiction configurations. Now, a new algorithm created by a team led by Daniel Tamayo is being set loose to try and figure out what systems are and are not stable. According to Tamayo: Separating the stable from the unstable configurations turns out to be a fascinating and brutally hard problem.
To make sure a planetary system is stable, astronomers need to calculate the motions of multiple interacting planets over billions of years and check each possible configuration for stability – a computationally prohibitive undertaking.
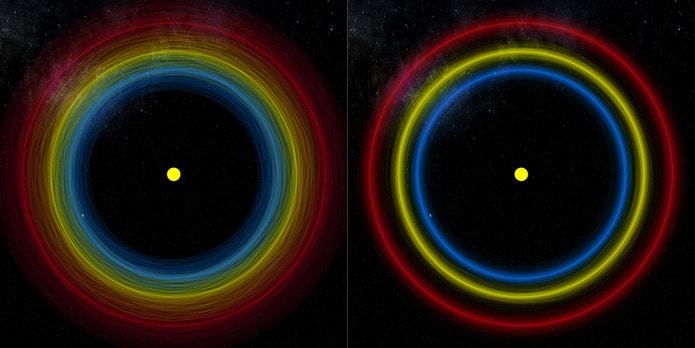
Rather than brute force their models, this new software samples how a system behaves over 10,000 orbits and looks for characteristic resonances and other dynamical characteristics that may predict instabilities. They then use artificial intelligence (AI) that has been trained to know what unstable systems look like to determine if the observed system will become unstable. They estimate this speeds up the modeling process by a factor of 100,000.
Machine learning algorithms like this one aren’t always doing what we think they’re doing, so there is still a need to run brute-force models now and then to try and verify this system’s predictions. Still, this is a start and it provides guidance on what systems can broadly be ignored as not stable, and which we expect to see over and over throughout the galaxy.
Before we end this episode, I just want to remind you that this weekend is our first of what we will be an annual CosmoQuest-a-Con event. Tickets are on sale, starting at just $20, and our vendors are all offering either discounts or a free item with purchase. This means your $20 ticket can get you a 10% discount from our friends at Oceanside Photo and Telescope, or a chance to win equipment donated by Explore Scientific as prizes for our contests.
Learn More
Gamma-Ray Burst Leaves Most Distant Optical Afterglow
- Northwestern University press release
- “Discovery of the Optical Afterglow of Short GRB 181123B at z = 1.754: Implications for Delay Time Distributions,” Kerry Paterson, Wen-fai Fong et al., 2020 July 14, Astrophysical Journal Letters (Preprint on arxiv.org)
Gigantic, Red, and Full of Spots
- Max Planck Institute for Solar System Research news release
- “Active Red Giants: Close Binaries Versus Single Rapid Rotators,” Patrick Gaulme, Jason Jackiewicz, Federico Spada et al., 2020 July, Astronomy & Astrophysics (Preprint on arxiv.org)
Massive Stellar Embryos Undergo Episodic Growth
- Max Planck Institute for Astronomy news release
- “New Maser Species Tracing Spiral-Arm Accretion Flows in a High-Mass Young Stellar Object,” Xi Chen et al., 2020 July 13, Nature Astronomy (Preprint)
Artificial Intelligence Predicts Which Planetary Systems Will Survive
- Simons Foundation news release
- “Predicting the Long-Term Stability of Compact Multi-Planet Systems,” Daniel Tamayo et al., 2020 Proceedings of the National Academy of Sciences
Credits
Written by Pamela Gay
Hosted by Pamela Gay
Audio and Video Editing by Ally Pelphrey
Content Editing by Beth Johnson
Intro and Outro music by Kevin MacLeod, https://incompetech.com/music/