Fast, strong magnetic winds caused by quickly rotating pulsars may be accelerating particles like electrons to extremely high-energy states and creating gamma-ray photons in their wake. Plus, missions close to home, large and distant objects, some pretty Hubble photos, and laser simulations of fast radio bursts.
Podcast
Show Notes
Students study magnetic fields from ISS
- High school students measure Earth’s magnetic field from ISS (EurekAlert)
- “Modelling the Earth’s magnetic field,” Nuno Barros e Sá and Miguel Cymbron, 2022 May 23, American Journal of Physics
Magnetic waves discovered in Earth’s core
- ESA press release
- “Satellite magnetic data reveal interannual waves in Earth’s core,” Nicolas Gillet et al., 2022 March 21, PNAS
Lucy spacecraft spies lunar eclipse
- NASA Goddard press release
Another solar crisis averted
- MPIA press release
- “Observational constraints on the origin of the elements: IV. Standard composition of the Sun,” Ekaterina Magg et al., 2022 May 20, Astronomy & Astrophysics
FAST measures hydrogen absorption in planetary nebula
- Research sheds more light on the properties of young planetary nebula IC 4997 (Phys.org)
- “FAST search for circumstellar atomic hydrogen–I: the young planetary nebula IC 4997,” Xu-Jia Ouyang et al., to be published in The Astrophysical Journal (preprint on arxiv.org)
Glowing galaxies in infrared and ultraviolet
- NASA Goddard image release
Pulsars pack a photonic punch
- Pulsars may power cosmic rays with the highest-known energies in the universe (Science News)
- “On the Potential of Bright, Young Pulsars to Power Ultrahigh Gamma-Ray Sources,” Emma de Oña Wilhelmi, Rubén López-Coto, Elena Amato, and Felix Aharonian, 2022 April 28, The Astrophysical Journal Letters
- “Gamma-ray haloes around pulsars as the key to understanding cosmic-ray transport in the Galaxy,” Rubén López-Coto et al., 2022 February 14, Nature Astronomy
Using lasers in labs to understand Fast Radio Bursts
- PPPL press release
- “Collective plasma effects of electron–positron pairs in beam-driven QED cascades,” Kenan Qu, Sebastian Meuren, and Nathaniel J. Fisch, 2022 April 21, Physics of Plasmas
- “Signature of Collective Plasma Effects in Beam-Driven QED Cascades,” Kenan Qu, Sebastian Meuren, and Nathaniel J. Fisch, 2021 August 27, Physical Review Letters
Transcript
Today’s show is all about energy, from pulsars accelerating electrons to make gamma-rays to a student experiment measuring the Earth’s magnetic field from space using a Raspberry Pi.
We also have some pretty Hubble photos, including uncommon galaxies.
We conclude with lasers, not on sharks, but in California.
All that and more, right here on the Daily Space.
I am your host Dr. Pamela Gay.
And I am your host Erik Madaus.
And we’re here to put science in your brain.
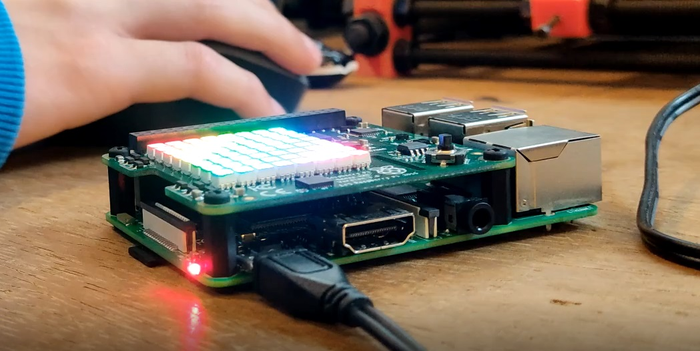
The Raspberry Pi mini-computer has allowed students and others to get experience in programming and building electronics. Amateur astrophotographers also use the small computers to automate their telescopes. And ESA’s AstroPi project recently sent one of them into space to the International Space Station. ESA put together a competition to get student experiments to run on the in-space hardware, and the program’s first results are out.
All of the student experiments used the same hardware, called the Sense Hat. The students from a high school in Portugal programmed the AstroPi for magnetic field research. The students got data from about three hours of International Space Station (ISS) orbits and were able to roughly map the whole Earth’s magnetic field. In their paper published in the American Journal of Physics, they compared their ISS results to a professional network of satellites and ground stations that does a survey every 5 years. This survey, the International Geomagnetic Reference Network, was last done in 2020.
And the students’ results were close to the professionals’, with a fixed variation likely caused by the local field of the station’s electronics. The students tried again with fifteen more orbits of data and got better results.
According to the paper, the student project could be repeated on the ground. It doesn’t even need to use the Pi platform but could be done with smartphones. Faculty mentor Nuno Barros e Sá said: Taking measurements around the globe and sharing data via the internet or social media would make for an interesting science project that could connect students in different countries.
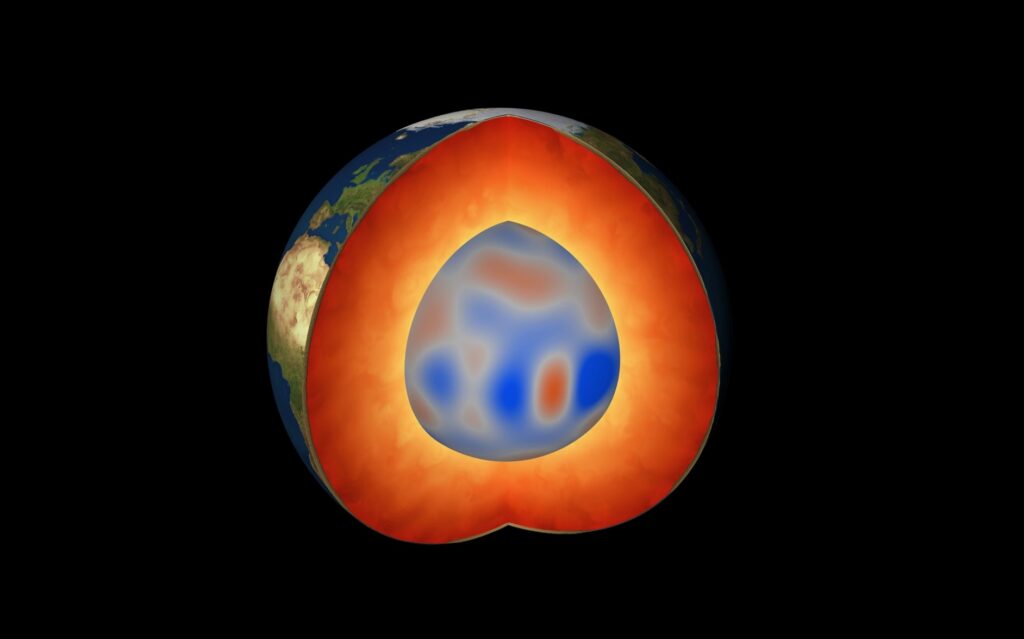
Mapping out our magnetic field, and understanding how it interacts with solar particles, is science that basically helps keep our civilization alive. After all, we’re pretty sure life owes a debt of gratitude to that magnetic field, which protects us from cosmic radiation and the solar winds. And that field is generated deep inside Earth, where the outer core of our planet is made of liquid iron, spinning and swirling, creating a dynamo.
Now, research published in the Proceedings of the National Academy of Sciences presents evidence of a newly discovered type of magnetic wave that runs along the outer edge of the core up against the mantle. The wave oscillates over a seven-year time frame and moves westward at 1,500 kilometers per year. Lead author Nicolas Gillet explains: Geophysicists have long theorized over the existence of such waves, but they were thought to take place over much longer time scales than our research has shown. Measurements of the magnetic field from instruments based on the surface of Earth suggested that there was some kind of wave action, but we needed the global coverage offered by measurements from space to reveal what is actually going on.”
That global coverage came from the European Space Agency’s Swarm satellites, a trio of identical satellites launched in 2013 for just this type of observation. The data collected was combined with measurements from two other European missions – Germany’s Champ and the Netherlands’ Ørsted – to create a computer model of Earth’s dynamo.
The team suspects that there are longer period waves to be found, but obviously, more observing time is necessary.
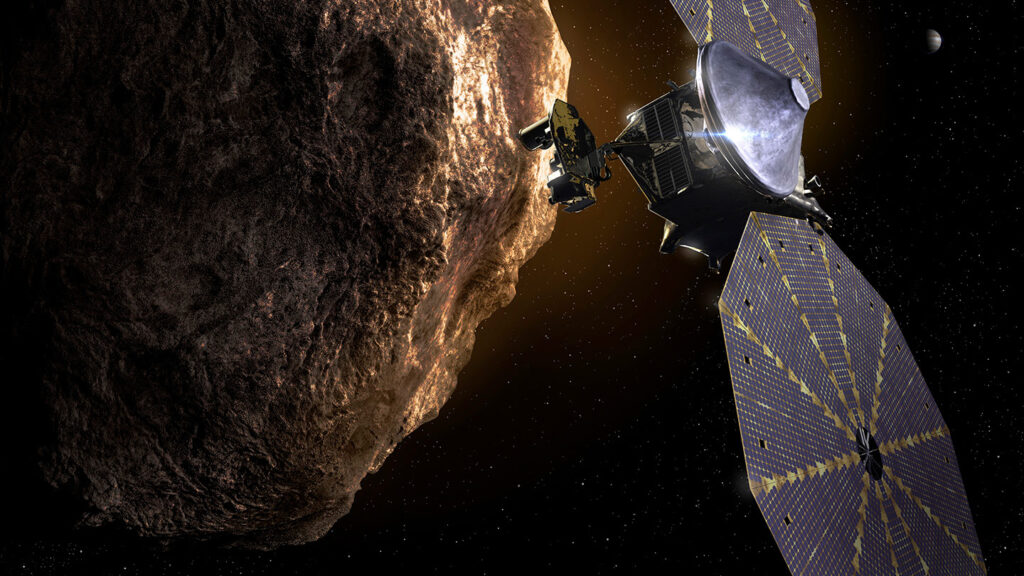
Swarm is doing what it was designed to do, but I’m always delighted when a spacecraft is used for observations outside its mission, proving how creative engineers and scientists can be given the opportunity. And earlier this month, the total lunar eclipse presented one spacecraft with a unique chance to view the event from space. And that’s just what NASA’s Lucy spacecraft did.
Lucy launched in October 2021 and is currently heading back toward Earth for another gravity assist this October to help sling the spacecraft out to Jupiter’s Trojan asteroids. This trajectory meant Lucy’s L’LORRI camera was able to capture the eclipse from about 100 million kilometers away.
Unfortunately, the timelapse video of the event is only about two seconds long. It’s definitely a “blink and you’ll miss it” clip. We’ll have a link to it in our show notes at DailySpace.org, so go take a moment to enjoy watching the Moon just sort of blip into darkness.
That’s enough with the latest in spacecraft missions. Let’s look at bigger objects like stars, planetary nebulae, and galaxies.
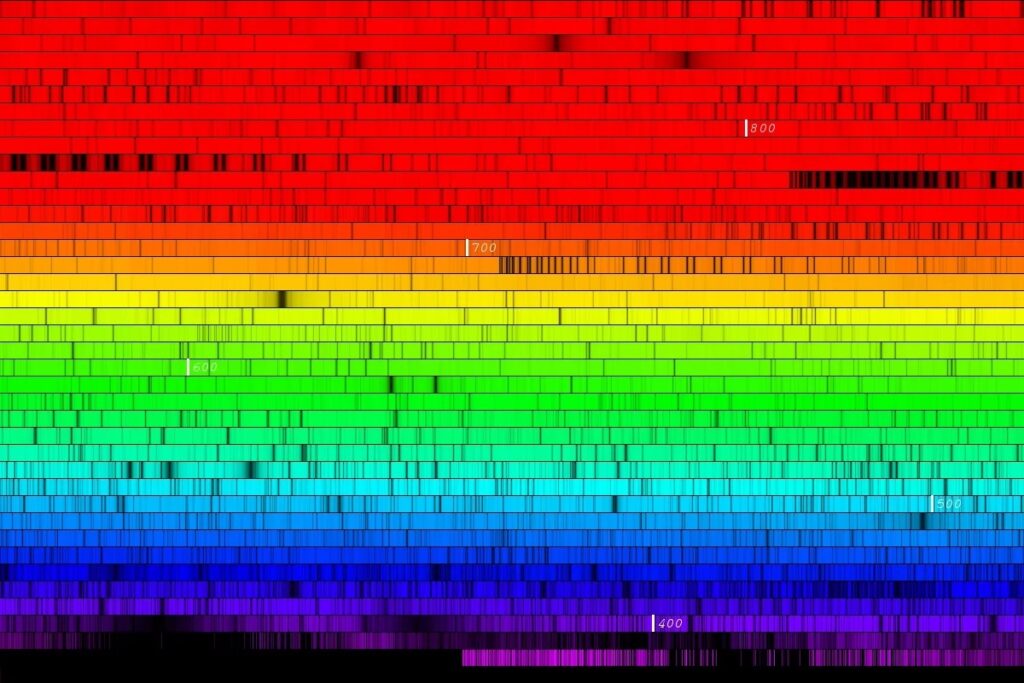
I would like to start this story by pointing out that solar scientists can be super dramatic in their naming. Astronomers – we’re just bad at it. But for solar scientists, everything is a crisis. I grew up hearing about the solar neutrino crisis, and with that solved, now I’m learning there is a solar abundance crisis. Back in 2009, the Sun’s chemical ingredients list was measured to a level of precision that should have made models of the Sun match what we see beautifully. But they didn’t.
The Sun is a mass of incandescent gas that shakes like liquid on a speaker playing a long low tone. We can see these waves reshaping the Sun’s surface, and the size of the waves is directly related to the volume of the part of the Sun that is resonating and the density of the material inside. This is like how the notes you can play when blowing in a soda bottle are related to the size of the empty part of the bottle, and if the bottle is filled with normal, mostly nitrogen gas or something else.
It turns out that those 2009 solar measurements predicted a Sun that should resonate with a different set of pitches than the ones our Sun’s surface is observed to actually play. And the solar scientists named this mismatch the “Solar Abundance Crisis” because it seemed to imply that our understanding of what is in the Sun’s atmosphere must not be right.
Again, they are dramatic in how they name things.
Turns out, the problem wasn’t in the measurements of the Sun’s ingredients. The problem was in our interpretation of those measurements.
In modeling how stellar atmospheres are heated and how they excite different atoms to absorb or emit light, it has generally been assumed the Sun is able to effectively spread heat through its atmosphere and is in what’s called thermal equilibrium. This implies that even though the Sun’s atmosphere is heated by roiling blobs of rising and falling gas that are all different temperatures, like the convecting blobs in a lava lamp, somehow the atmosphere can be modeled like everything – at least at the local level – is a nice smooth distribution of temperatures.
But in a new paper in Astronomy & Astrophysics with first author Ekaterina Magg, researchers in a team led by Maria Bergemann try modeling the Sun using calculations that don’t assume thermal equilibrium at local scales. This small change makes the maths a lot harder but also makes for a more realistic description of how energy moves in lower-density areas like the Sun’s photosphere.
And it turns out that these changes to the models changed what ratios of ingredients the Sun needs to have to produce the interrupted rainbow of light we see. According to Magg: The value for the oxygen abundance was almost 15% higher than in previous studies.
This change made the Sun better match both the compositions of primitive meteors that are representative of the early solar system and brought the results from the spectra and the helioseismology all into alignment
As they state in their press release: Crisis resolved.
Bergemann goes on to explain: The new solar models based on our new chemical composition are more realistic than ever before: they produce a model of the Sun that is consistent with all the information we have about the Sun’s present-day structure – sound waves, neutrinos, luminosity, and the Sun’s radius – without the need for non-standard, exotic physics in the solar interior.
At the end of the day, it seems it is a good idea to check your assumptions when maths and observations don’t match. Sometimes, our simplifications create false emergencies, and all it takes is a bit of more complex calculations to see things as they really are.
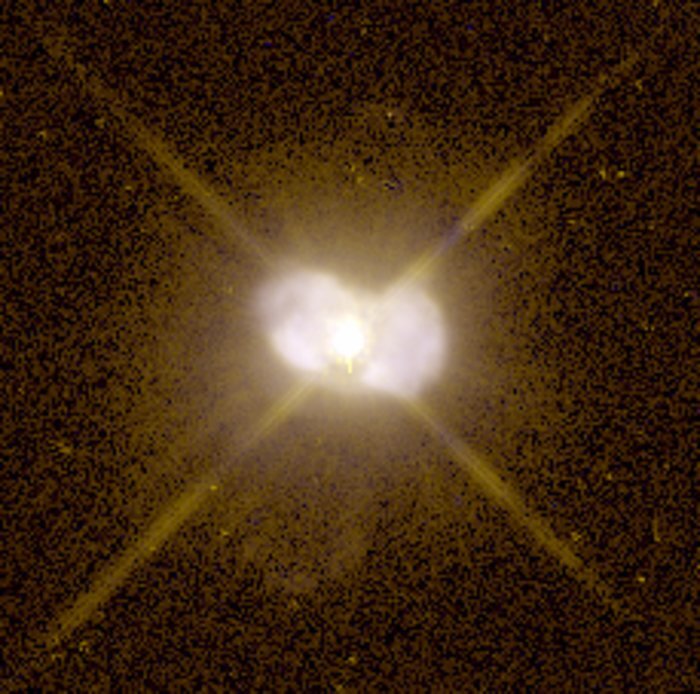
Our Sun is a main-sequence star. This means that sometime in the far distant future, about five billion years down the road, our star will puff up into a red giant. Even further down the road, it may blow off enough material to become a white dwarf. In the process, expanding shells of gas and dust might be ejected and form a planetary nebula, which would be gorgeous to see, if any of us were still around in our robot bodies to do so.
In the meantime, we have other planetary nebulae we can observe that can help us understand the chemical evolution of stars and even galaxies. A new paper on arxiv.org examines one such planetary nebula – IC 4997 – using the Five-hundred-meter Aperture Spherical radio Telescope (FAST) in China. This particular planetary nebula is about 8,000 light-years away in the constellation of Sagitta and has a relatively high surface brightness and bright central star. Additionally, IC 4997 is rapidly evolving, which makes it a great observational target.
Analyzing the radio data, scientists detected neutral atomic hydrogen absorption in IC 4997, which is a fancy way of saying there is cold hydrogen gas. And the atomic shell of the planetary nebula contains about 17 septendecillion hydrogen atoms (that’s 17 followed by 54 zeros), a number I never thought I would write let alone say. The evidence further suggests that this particular shell was ejected at least 1,000 years ago, but – and this is pretty common when it comes to planetary nebula – the nebular mass is still less than expected.
All of that is a long-winded way to say that the data collected is interesting but doesn’t solve the ongoing missing mass problem that seems to be common with planetary nebulae. However, FAST seems to be up to the challenge, and the team plans to look at more planetary nebulae in the near future.

Before we get into the heavy science in our final segment, here’s a pretty picture. The larger of the two galaxies in this picture, collectively called IC 4271, is a Seyfert galaxy. Named after Carl Seyfert, these are galaxies with a black hole in the center, making them glow brighter than other galaxies without black holes.
Specifically, the larger part of IC 4271 is a type 2 Seyfert because it glows in mostly infrared and optical light. This image is from a survey where astronomers looked at pairs of galaxies but only those where one is in front of the other as viewed from Earth. Hubble‘s Wide Field Camera 3 is capable of seeing ultraviolet through infrared wavelengths. In this image, ultraviolet light is colorized violet while near-infrared is shown in red.
Coming up, scientists continue to try and understand just how certain high-energy events work.
There are some research papers I read and realize I am really glad I’m not in grad school anymore because the paper I’m reading would be a homework problem. There are a remarkable number of problems in astronomy where lots and lots of folks have said “that could be X”, but no one really wanted to do the maths to figure it out. Then, once one person has done it, lots of folks assign what they did as homework, using their paper as the solution guide.
A remarkable amount of grad school was learning how to find the papers our homework problems were stolen from. I am still bitter about the homework problem that came from a paper that resulted in the original researcher getting a Nobel Prize.
Anyway…
A pair of new papers in The Astrophysical Journal Letters and Nature Astronomy, led respectively by E. de Oña Wilhelmi and R. López-Coto, looks at the regions around young neutron stars and asks the simple question: Are the magnetic fields sufficient to accelerate electrons to the kinds of speeds necessary for them to produce gamma-ray light when they collide with photons?
They did this exercise specifically to prove that this is not what was happening, but the math replied with “well, actually…“, and they found themselves proving that yes, the magnetic fields around young stars are that strong.
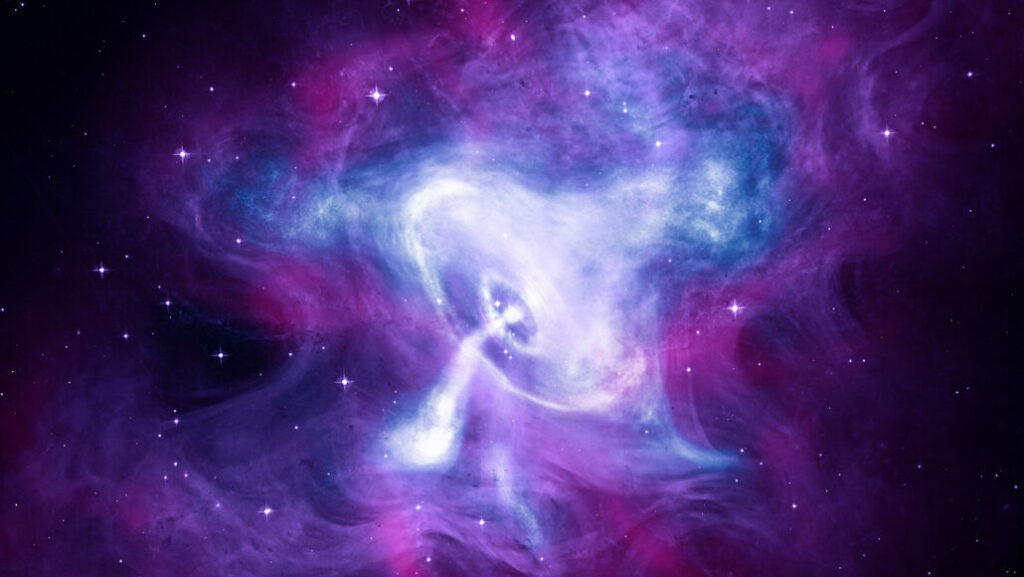
Here is what is happening. As the young neutron stars – less than 200,000 years old – spin, they generate powerful magnetic fields. Their surroundings aren’t exactly empty. Neutron stars are the leftovers of massive stars that had previously undergone all sorts of mass loss, and the young objects are extremely hot and produce ionizing radiation that can separate electrons from their atoms. Those electrons, in the powerful magnetic field, get accelerated like electrons in the large hadron collider, just more so.
While some of these electrons will eventually reach Earth as cosmic rays, their paths are hard to trace because they are affected by intervening magnetic fields from other stars and black holes. Some of these electrons, however, interact with photons in the vicinity of the pulsar and pump the energy of the photons to the gamma-ray part of the spectrum. These extremely high-energy bits of light then fly to Earth on straight lines that ignore magnetic fields. We knew that pulsars had gamma rays, but the why of it wasn’t detailed out prior to these papers.
Now, we have a nice, consistent answer to both where some cosmic rays come from and why pulsars produce gamma rays. It is a beautiful result, and I’m really glad I don’t have to replicate their math.
Sometimes, the work being done in labs is astounding, to say the least. And when you find out that the experiments involve high-power lasers, well, how can you resist reading more?
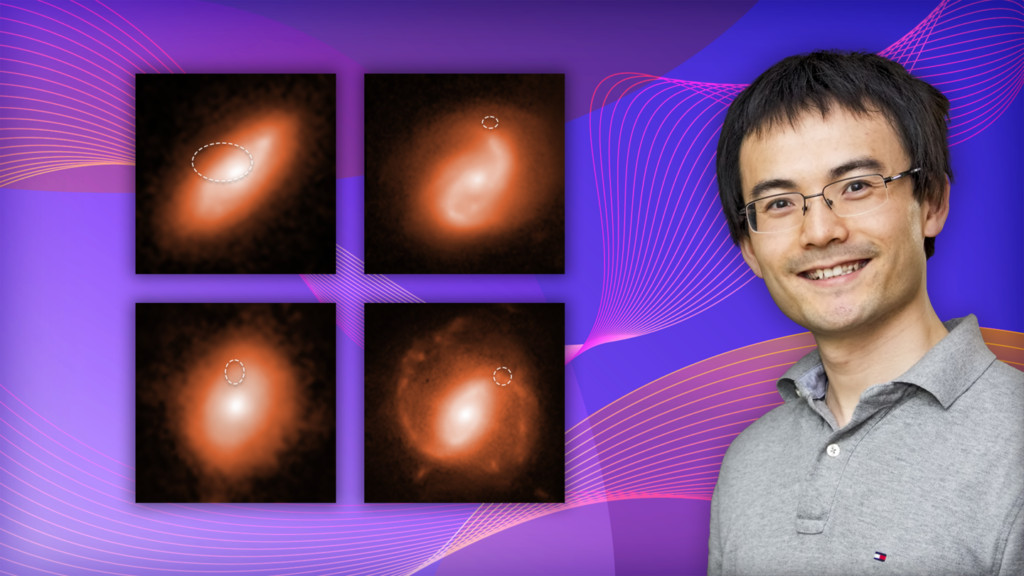
Scientists from Princeton University, the U.S. Department of Energy’s (DOE) Princeton Plasma Physics Laboratory (PPPL), and the SLAC National Accelerator Laboratory have found a way to simulate the high energy of a magnetar in the lab. They’re doing this to get at understanding what fast radio bursts are and how they happen. Their research was published in two journals – Physics of Plasmas and Physical Review Letters – and was led by Kenan Qu from Princeton.
Magnetars are neutron stars contained within incredibly strong magnetic fields that basically convert the vacuum of space into a strange matter-antimatter plasma, or ‘pair plasma’. This particular soup is different from the plasma of the Sun because it only has electrons and higher-mass ions, and of course, that leads to strange behavior.
Creating this particular type of plasma in the lab requires not using magnetar strength magnetic fields but rather lasers. As Qu explains: Rather than simulating a strong magnetic field, we use a strong laser. It converts energy into pair plasma.. [which] then shifts the laser pulse to a higher frequency. The exciting result demonstrates the prospects for creating and observing…pair plasma in laboratories and enabling experiments to verify theories about fast radio bursts.
Before anyone gets too excited, Qu notes: No lasers are strong enough to achieve this today, and building them could cost billions of dollars. Our approach strongly supports using an electron beam accelerator and a moderately strong laser to achieve QED pair plasma. The implication of our study is that supporting this approach could save a lot of money.
The simulation is expected to be tested at SLAC in the near future, and we look forward to hearing more about this fascinating work.
This has been the Daily Space.
You can find more information on all our stories, including images, at DailySpace.org. As always, we’re here thanks to the donations of people like you. If you like our content, please consider joining our Patreon at Patreon.com/CosmoQuestX.
Credits
Written by Pamela Gay, Beth Johnson, Erik Madaus, and Gordon Dewis
Hosted by Pamela Gay, Beth Johnson, and Erik Madaus
Audio and Video Editing by Ally Pelphrey
Content Editing by Beth Johnson
Intro and Outro music by Kevin MacLeod, https://incompetech.com/music/