An analysis of the thickness and the shapes of the ice mounds in Martian craters found that the patterns matched Mars’ axial tilt and precession over the last 4 to 5 million years. Plus, Europa, faint galaxies, What’s Up, and a review of an entire camera.
Podcast
Show Notes
Craters have lasting impact on planetary research
- Purdue press release
- “Earth Impact Effects Program: A Web-based computer program for calculating the regional environmental consequences of a meteoroid impact on Earth,” Gareth S. Collins, H. Jay Melosh, and Robert A. Marcus, 2010 January 26, Meteoritics & Planetary Science
Ice mounds abound in Martian craters
- AGU press release
- “Orbital Forcing of Martian Climate Revealed in a South Polar Outlier Ice Deposit,” Michael M. Sori et al., 2022 March 29, Geophysical Research Letters
Europa’s chaos terrains could support life?
- UT press release
- “Downward Oxidant Transport Through Europa’s Ice Shell by Density-Driven Brine Percolation,” Marc A. Hesse et al., 2022 February 10, Geophysical Research Letters
Long orbits make long bars
- AIP press release
- “The VMC survey – XLVI. Stellar proper motions in the centre of the Large Magellanic Cloud,” Florian Niederhofer et al., 2022 March 29, Monthly Notices of the Royal Astronomical Society
Hiding galaxy teased out of the sky
- Astronomers discover a new ultra-faint dwarf galaxy (Phys.org)
- “Pegasus IV: Discovery and Spectroscopic Confirmation of an Ultra-Faint Dwarf Galaxy in the Constellation Pegasus,” W. Cerny et al., submitted to AAS journals (preprint on arxiv.org)
What’s Up: Algol
- Algol is the Demon Star (EarthSky)
- John Goodricke (Sky Over Berlin via Internet Archive)
- “Dimensions of the Fixed Stars, with Especial Reference to Binaries and Variables of the Algol Type,” Edward C. Pickering, 1880 May 25, Proceedings of the American Academy of Arts and Sciences
- “A Spectroscopic Investigation of Algol,” Alan S. Meltzer, 1957 March, Astrophysical Journal
- “Two centuries of study of Algol systems,” Alan H. Batten, 1989 June, Space Science Reviews
Transcript
Today is a day of violence and hiding. Not by us. We’re fine.
But we have tales of planets getting cratered by incoming asteroids and the ice mounds that can grow in those craters.
And we have faint galaxies trying really hard to hide in the outskirts of the Milky Way.
They did not succeed in hiding, by the way.
But we’re fine. The skies are nice, a bit filled with aurorae, and there are variable stars waiting to be watched.
We’ll talk about all of this and more, right here on the Daily Space.
I am your host Dr. Pamela Gay.
And I am your host Erik Madaus.
And we’re here to put science in your brain.
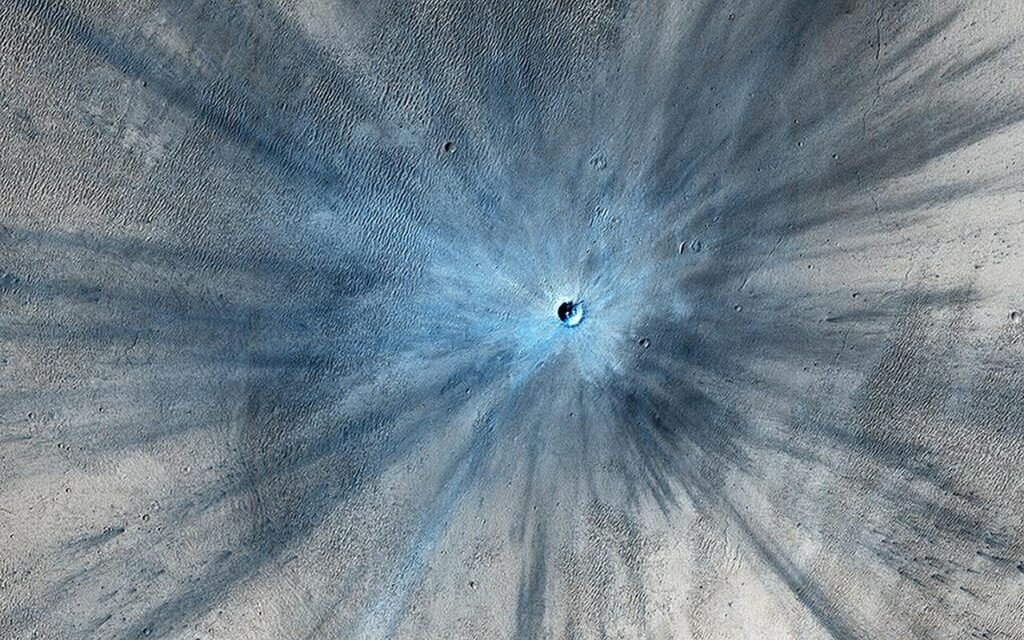
First up, things that go bump and crash. Honestly, the best open to this story is the one in the press release: While for humans the constants might be death and taxes, for planets the constants are gravity and collisions.
And the great thing about those collisions, so long as we’re not personally experiencing them, is that they can be used to understand the history, formation, and evolution of our solar system and many of the various solid bodies within it. I say solid bodies because, while impacts at Jupiter are cool, they don’t exactly leave a lasting impression. Rest in peace, Comet Shoemaker-Levy 9.
So how do we go about studying all these widely varied impact craters? Enter Brandon Johnson from Purdue University, who collects impact data from various missions and labs and then works with his team to build models that help us understand what happened, when, and just what all that stuff out there is made of. And it’s not just about other solar system bodies, either. Johnson also works on craters on the Moon and even here on Earth.
Johnson explains just why he studies impact craters and meteorites: Most meteorites contain chondrules — small, previously molten, particles. Essentially, by studying the formation of chondrules by impacts, we can better understand what was going on in the nascent solar system. For example, based on one impact, we were able to determine that Jupiter had already formed right around 5 million years after the first solar system solids, changing the timeline of our understanding of the solar system.
Not only does Johnson have his own lab working on these studies, but he is also working to update an online impact calculator tool that was developed by his late mentor, Jay Melosh. Johnson and his team are rebuilding this online tool so that the next generation of planetary scientists can use it to understand how impacts affect Earth’s crust, atmosphere, and biosphere.
We look forward to the research coming from Dr. Johnson’s lab in the future.
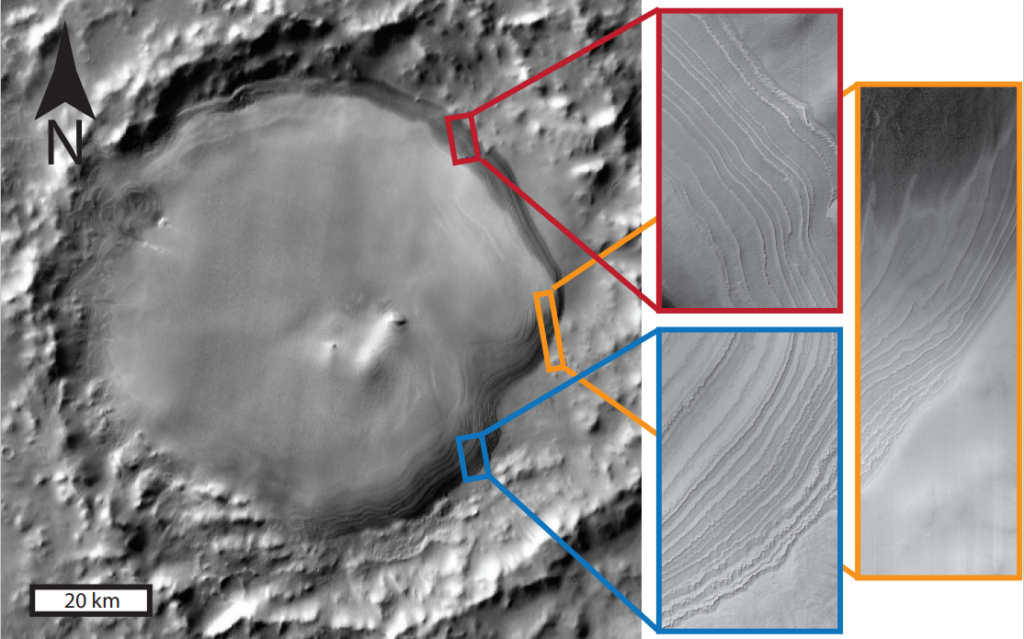
Speaking of using craters to understand the history of our solar system, researchers recently discovered deposits of layered ice in craters in Mars’ southern hemisphere. These deposits gave the team insights into the past orientation of the planet, which in turn, helps us understand more about Mars’ past climate. And that, of course, can help us figure out just when Mars could have been habitable.
The results of this study were published in Geophysical Research Letters with lead author Michael Sori, who matched the ice layers to the tilt of Mars’ axis. Basically, these ice deposits tell us about the temperature, hydrology, and even planetary dynamics of the red planet, just as similar layers can tell us about the same aspects of Earth. The tilt of a planet and how it orbits the Sun affects the temperature which depends on the amount of sunlight hitting the surface.
So thicker layers mean colder periods. That makes sense, right?
You also have to add in the precession of the planet’s axis, or how much it wobbles in space like a top. That can also affect how much sunlight reaches the surface, and as the precession changes the axial tilt over time, different regions are affected in different ways and at different times. While this research only applies to Mars’ recent past, it does come with an unprecedented amount of resolution. Sori notes: It was unexpected how cleanly those patterns matched to the orbital cycles. It was just such a perfect match, as good as you can ask for.
Sori and his team used one crater in particular, Burroughs crater, which is 74 kilometers wide and has “exceptionally well-preserved” ice layers that were visible in imagery taken by the HiRISE camera onboard NASA’s Mars Reconnaissance Orbiter. An analysis of the thickness and the shapes found that the patterns matched that axial tilt and precession over the last four to five million years.
It should be noted that one of the requirements for the ice layers they used in the study was that they had to be young and clean, which is why we currently cannot look much farther back in Mars’ history. Older ice is more complicated to study with a lot of variables to account for. It can be done, but it’s not as precise as scientists prefer.
Sori mentions that a welcome area of research would be if we ever can get ice cores on Mars as we do on Earth. Our rovers currently aren’t capable of that, so for now, we’ll have to stick with ground-penetrating radar and high-resolution imagery.
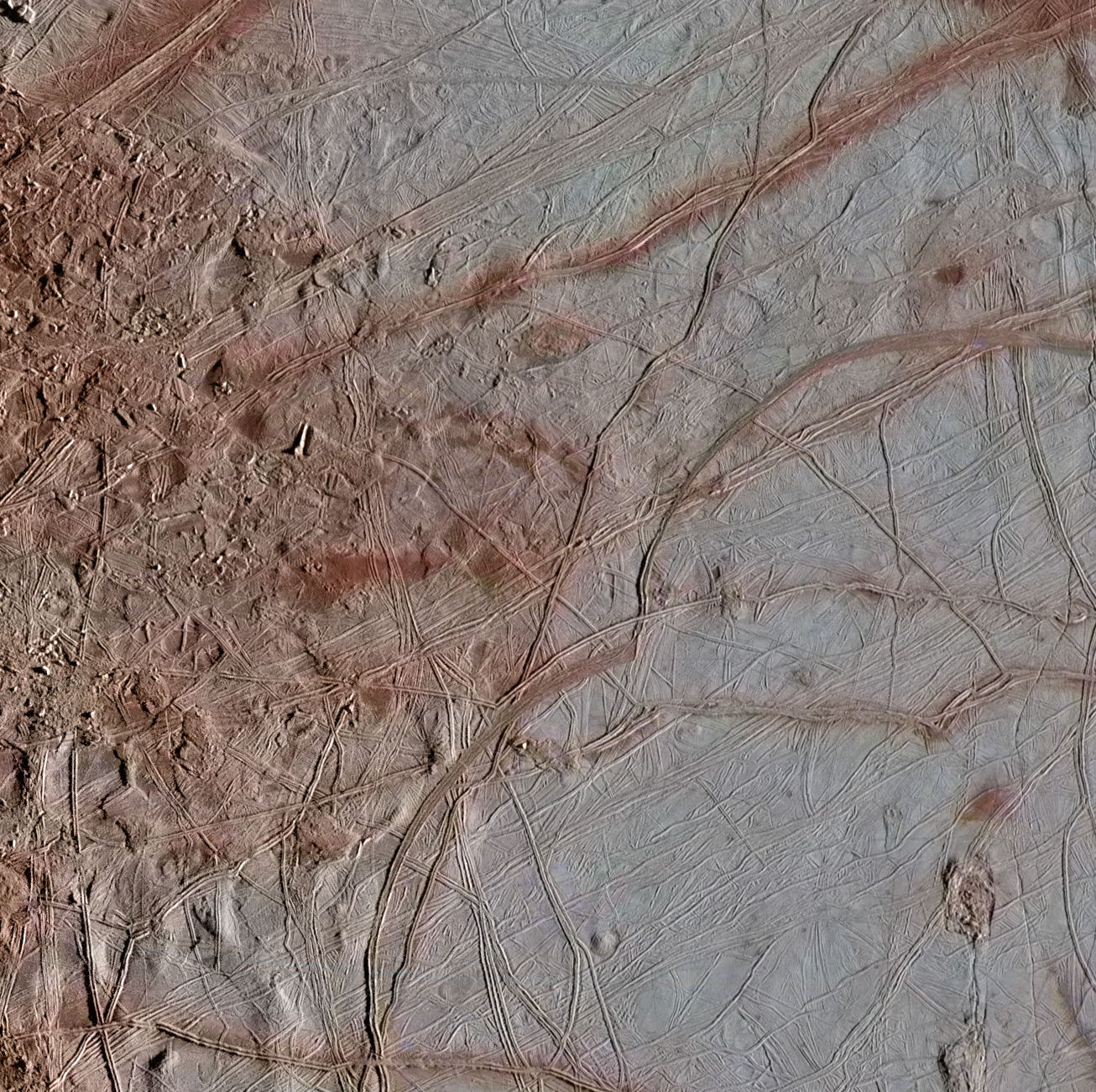
And of course, what would a section on impacts and ice be without some new research on Jupiter’s moon Europa? A new study published in Geophysical Research Letters finds that the chaos terrains of Europa – broken up, cracked, ridged regions – may be moving oxygen into the subsurface ocean.
Here’s how this could work. Sunlight and charged particles from Jupiter hit the icy shell of Europa, creating water and oxygen molecules. Those molecules can then mix with partially melted surface brines, or saltwater, which is then moved through the cracks of the chaos terrains. Some of those cracks can actually make their way deeper into the icy shell, depositing the briny water into the subsurface ocean and bringing oxygen with it.
And that oxygen is good for life. In fact, the model used in this study found that 86% of the oxygen taken in at the surface can make its way to the ocean. Add the long life of our solar system into the mix, and there could be plenty of oxygen in the subsurface oceans to support a lot of life. As co-author Steven Vance notes: It’s enticing to think of some kind of aerobic organisms living just under the ice.
Yes. Yes, it is. So, when does Europa Clipper launch again?
Coming up next, we’ll take a look at some galactic science, including a fainter than faint fuzzy.
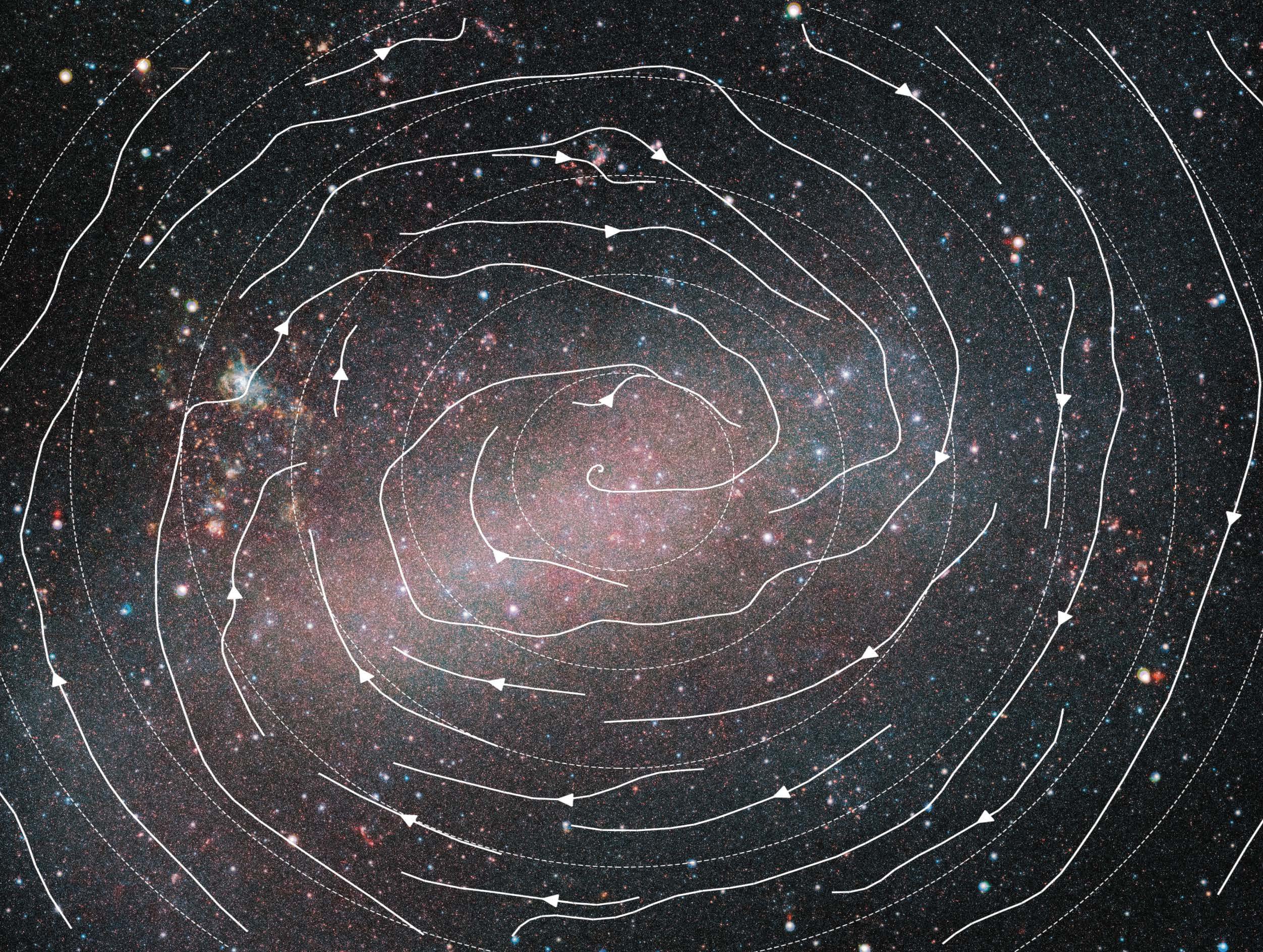
When most of us think of spiral galaxies, we think of beautiful systems of spiraling stars that are all sweeping arcs tracing out the golden ratio in stars. The thing is that there are a lot of galaxies with interrupting bars that stick out like sprinkler heads that disrupt the normal grace of these systems.
Exactly why some galaxies have bars is still not really understood, but one thing we do know is that bars aren’t unique to spirals like our Milky Way, which does have a bar, and we think this structure has something to do with companion galaxies. We actually see a bar in the nearby Large Magellanic Cloud, which is so nearby that we can measure the velocities of individual stars.
This creates a powerful opportunity to get at the physics behind these structures, and taking advantage of this opportunity is a team of researchers at Leibniz Institute for Astrophysics using data from the VISTA survey of the Magellanic Clouds system. According to principal investigator Maria-Rosa Cioni: Thanks to their close proximity of about 163,000 light-years, we can observe individual stars within the Magellanic Clouds using ground-based telescopes like VISTA. Thus, these galaxies provide us with a unique laboratory to study in great detail the processes that shape and form galaxies.
And using this data, they found the specific family of elongated orbits behind the visible bar structure.
This work is described in a new paper in Monthly Notices of the Royal Astronomical Society with lead author Florian Niederhofer, who explains: A specific type of these [elongated orbits] are the ones that are aligned with the major axis of the bar. These are considered to be the ‘backbone’ of stellar bars and provide the main support of the bar structure.
Getting at the orbits that build up the bar wasn’t an easy observation. This team used nine years of observations to get this result, and it was only possible because the Magellanic clouds are essentially in the outer suburbs of our Milky Way. Niederhofer goes on to explain: Our discovery provides an important contribution to the study of dynamical properties of barred galaxies since the Magellanic Clouds are at present the only galaxies where such motions can be investigated using stellar proper motions. For more distant galaxies this is still beyond our technical capabilities.
This just goes to show that sometimes, when you’re trying to understand the universe, you need to just take a really good look at what is in your own local neighborhood.
Understanding the Magellanic Clouds can be hard, and considering they are visible to the unaided eye in the Southern Hemisphere, that should put in perspective how much harder understanding more distant, smaller, and fainter systems can be.
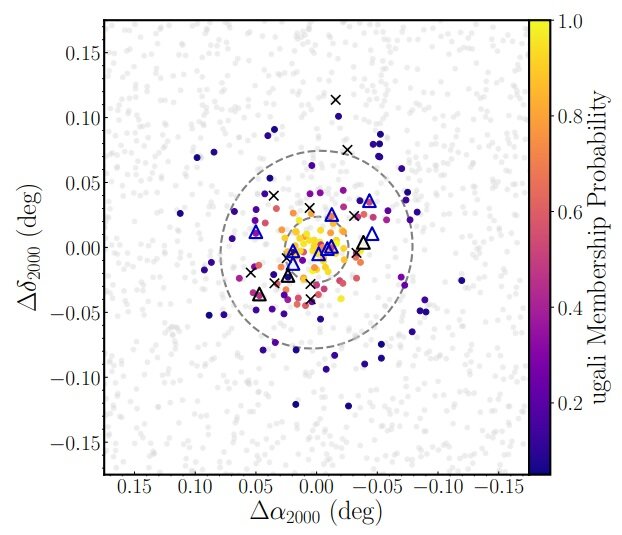
One of the more annoying kinds of systems out there is the ultra-faint dwarf galaxy. These systems are bound together by a proportionally large amount of dark matter that contains wisps of stars and gas that are just hard to see. They contain so little material capable of forming stars that they have had very few generations of stars and are essentially fossilized in a metal-poor state. This atomic simplicity makes them particularly interesting as they can help us understand the chemical evolution of galaxies early in the universe.
But to use them you have to find them, and they like to hide. They are so hard to find that we are still finding them in the outskirts of our Milky Way.
In a new paper appearing on arxiv.org and led by W. Cerny, a team highlights a newly found, ultra-faint dwarf named Pegasus IV that is right there, shining faintly, in the fall and winter sky. Located just 293,000 light-years away, this dwarf galaxy has about 4,400 solar masses of stars with ages of up to at least 12.5 billion years. This is a small, old system that is close enough for us to see individual stars.
And this won’t be the last system like this we are likely to find. With each new telescope survey, we can expect to find a few more of these systems waiting to have their member stars teased out of the background of our galaxy’s light.
One of the awesome things about dwarf galaxies is that, for the more luminous ones, you can actually image them with larger off-the-shelf telescopes and play “do you see the galaxy?” with friends. They are nothing more than areas with a few more stars, and it is amazing to realize these systems have entirely their own history that can often be revealed by looking at their variable stars.
And, if you’ve never looked at variable stars, Erik has a treat for you in this week’s What’s Up.
What’s Up

Algol is a star in the constellation Perseus. For centuries humans have known something was up with it. In Arabic, it was called the “demon star”. In the pseudoscience of astrology, it is associated with violence, and historical generals tried to avoid having battles while Algol was at a minimum. It increases and decreases in brightness a noticeable amount over a period of just under three days, making it a variable star. If you have a run of good clear nights, you can see this cycle yourself with the unaided eye.
Its variability was first written about in the seventeenth century, but it took over one hundred years for someone to propose a mechanism for its observed change in brightness in 1783. This explanation was almost right, which is impressive for back then. British astronomer John Goodricke proposed that there was something dark that regularly passed in front of the star, making it appear dimmer. The correct answer wasn’t far off, but it would take yet another century to prove it.
In 1881, American astronomer Charles Pickering discovered that there was another star orbiting Algol, which was dimmer than the main star and which he called an eclipsing binary star. The orbit of the fainter star caused the regular apparent change in brightness. This was discovered purely by analyzing the Doppler shift of the two stars and noticing that they weren’t what they were supposed to be. This 1881 work wasn’t the end, however, because the doppler data revealed some things that couldn’t be explained by just two stars.
In 1957, a third star in the system was discovered, and in 2019, NASA’s TESS spacecraft narrowed the lightcurve period down to the minute. For the nerds: two days, twenty hours, 49 minutes.
To find Algol, look for the “W” asterism of Cassiopeia in the northwest. To the west of Cassiopeia is Perseus, where Algol is. Perseus has a line of stars with two more below and to the left. Algol is one of these lower two. Algol is also halfway between the bright star Aldebaran in Taurus and below Capella in Auriga.
Next up, I’m going to review an actual camera and not just a lens.
Review

Instead of a lens review this week I’m going to be reviewing a camera body, the Canon EOS RP. The RP was my first Canon camera, and I found it really easy to use. I used this camera for over a year and shot about fifteen thousand pictures on it, so I think I’m qualified to review it.
Released in March 2019, the Canon EOS RP was Canon’s second full-frame mirrorless camera in the R series. It uses the new RF mount. The RP uses the 26.2-megapixel sensor from the 6D mark 2. A new body costs $999, or $899 for a refurbished one from Canon that includes a one-year warranty.
Canon markets this as the smallest and lightest full-frame EOS camera. It is 132 millimeters long and 85 millimeters tall. It weighs 485 grams including the battery and an SD memory card. Canon sells a small “extension grip” to make the bottom of the camera about a centimeter thicker. I had one as part of my RP kit and really did not like it. It didn’t add enough length to be useful and made it difficult to remove the battery and memory cards. The rest of the camera was relatively comfortable to hold. I loved the overall size because it allowed me to put it in my jacket pocket with a nifty fifty lens on it.
The RP uses the LP-E17 battery found on the Rebel series of DSLRs. This gives it a maximum rating of 210 shots per battery using just the electronic viewfinder; less if you use both the electronic viewfinder and the rear screen. However, in real conditions, I managed to get as many as 1,600 on one battery but usually five to six hundred, using primarily the viewfinder.
There are some things about the RP to be aware of if you’re thinking of buying one. It’s a mirrorless camera, which means you look into a small screen showing what the sensor sees instead of a mirror, so what you see through the viewfinder will be ever so slightly behind real-time, which can make tracking fast-moving objects tricky; something other more expensive R-series models are better at.
The electronic viewfinder is easy to use, and a neat feature offered by it is exposure simulation. Essentially, the camera can show you what the final image will look like before you press the shutter. Exposure simulation pretty much eliminated me looking at the rear screen after each photo. It’s also a good feature for beginning photographers, as you can see what changing different exposure settings does to the image in near real-time.
A lot of the buttons are customizable via the menus, but not all buttons can be reassigned.
You can access the menus via the EVF or the rear screen, so most of the time I had the rear screen closed. This has led to some weird looks from people as I appeared to intently focus on something at my feet with my giant 600mm lens when I was actually looking at the menus.
The RP’s sensor offers plenty of megapixels, and while it suffers a little in dynamic range, it has decent high ISO performance, up to about 12,800, above which it starts to get a bit noisy. I have no problems with its image quality.
Most of the native RF mount lenses are expensive, but you can use any EF lenses that you may already have on the RP using an adapter. I’ve used EF lenses on the RP, and they perform brilliantly. A lot of people online say that their EF lenses work better adapted to RF mount cameras. It can also mount EF-S lenses designed for crop sensors, though this cuts the resolution down to about ten megapixels.
Another feature I liked in this camera is focus bracketing. You tell the camera how many pictures to take and by how much to move the focus in between, and it takes them. When it judges it has reached the back of the scene, it stops taking photos. Then you stack these photos on a computer using free Canon software to produce a final image that is in focus from front to back. This is particularly useful for macro photography and I was surprised to find it in a camera this inexpensive.
In its standard mode dial, the RP has three custom functions available, which I used for my wildlife photography because they allowed me to quickly change settings in response to different situations.
Like most cameras these days, it can also take videos; however, I didn’t explore this, so I can’t really comment on it.
To sum things up, I think the Canon RP is a great beginner’s camera. It’s an inexpensive way to buy into the RF lens system, which has fantastic enthusiast lenses like the 600mm f/11 and 16mm f/2.8. I would recommend it for most purposes except perhaps for capturing birds in flight, as it isn’t quite up to that.
My full review of the RP was about twice as long as I could put in the show, so please read the full version on my Medium. The link to that will be in the show notes.
This has been the Daily Space.
You can find more information on all our stories, including images, at DailySpace.org. As always, we’re here thanks to the donations of people like you. If you like our content, please consider joining our Patreon at Patreon.com/CosmoQuestX.
Credits
Written by Pamela Gay, Beth Johnson, Erik Madaus, and Gordon Dewis
Hosted by Pamela Gay, Beth Johnson, and Erik Madaus
Audio and Video Editing by Ally Pelphrey
Content Editing by Beth Johnson
Intro and Outro music by Kevin MacLeod, https://incompetech.com/music/