The inner solar system was a wild and wooly place as the planets were forming, and new research shows that the collisions that formed Earth and Venus were likely of the hit-and-run variety. Plus, polar ice loss warps the planet, and a black hole eats a star.
Podcast
Show Notes
La Palma volcanic eruption reaches Atlantic Ocean
New process cooks water/oxygen from Moon rocks
- EPSC press release
- “Water production from lunar regolith through carbothermal reduction modelling through ground experiments,” Michelle Lavagna et al., 2021 September 13-24, European Planetary Science Congress 2021
Chang’e-5 lunar sample contains “exotic” material
- EPSC press release
- “The Exotic Materials at the Chang’e-5 Landing Site,” Yuqi Qian et al., 2021 September 13-24, European Planetary Science Congress 2021
Hydrogen used to find sub-surface water ice on Mars
- EPSC press release
- “Looking for Non-Polar Shallow Subsurface Water Ice in Preparation for Future Human Exploration of Mars,” German Martinez et al., 2021 September 13-24, European Planetary Science Congress 2021
Polar ice loss warps the shape of Earth
- Harvard University press release
- “The Global Fingerprint of Modern Ice-Mass Loss on 3-D Crustal Motion,” Sophie Coulson et al., 2021 August 16, Geophysical Research Letters
Cosmic billiards leads to inner planetary growth
- The University of Arizona press release
- “Collision Chains among the Terrestrial Planets. II. An Asymmetry between Earth and Venus,” Alexandre Emsenhuber et al., 2021 September 23, The Planetary Science Journal
Black holes shreds star, enabling mass measurement
- The University of Arizona press release
- “Mass, Spin, and Ultralight Boson Constraints from the Intermediate-mass Black Hole in the Tidal Disruption Event 3XMM J215022.4–055108,” Sixiang Wen, Peter G. Jonker, Nicholas C. Stone, and Ann I. Zabludoff, 2021 September 6, The Astrophysical Journal
Transcript
Hello and welcome to the Daily Space. I am your host Dr. Pamela Gay.
And I am your host Beth Johnson.
And we are here to put science in your brain.
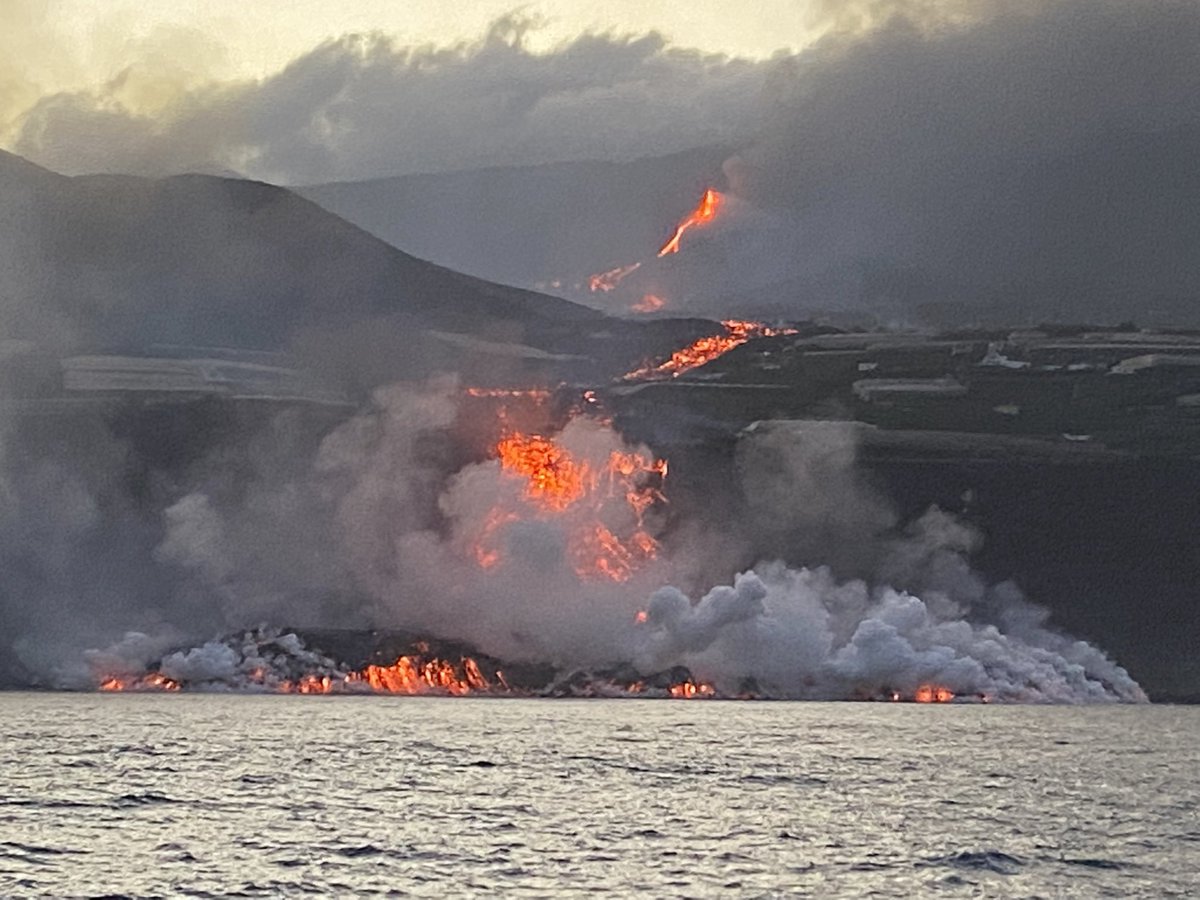
We would like to start today with a quick update on the volcanic eruption on the Island of La Palma in the Canary Islands. After eight days of seismic activity, the La Palma volcano erupted from a series of new fissures on September 18 and has been flowing ever since. There was a brief pause on September 27 before the eruption resumed, and late on the night of September 28, the lava flows reached the Atlantic Ocean. There have still been no injuries or deaths thanks to the combined efforts of the local government and scientific community; however, over 650 structures and 20 kilometers of road have been destroyed. So long as people remain safe, we’ll continue to livestream the eruption in between our regularly scheduled shows.
And now for the rest of the news.
The Europlanet Science Congress 2021 wrapped up on Friday, September 24, and we have a few more press releases to cover.
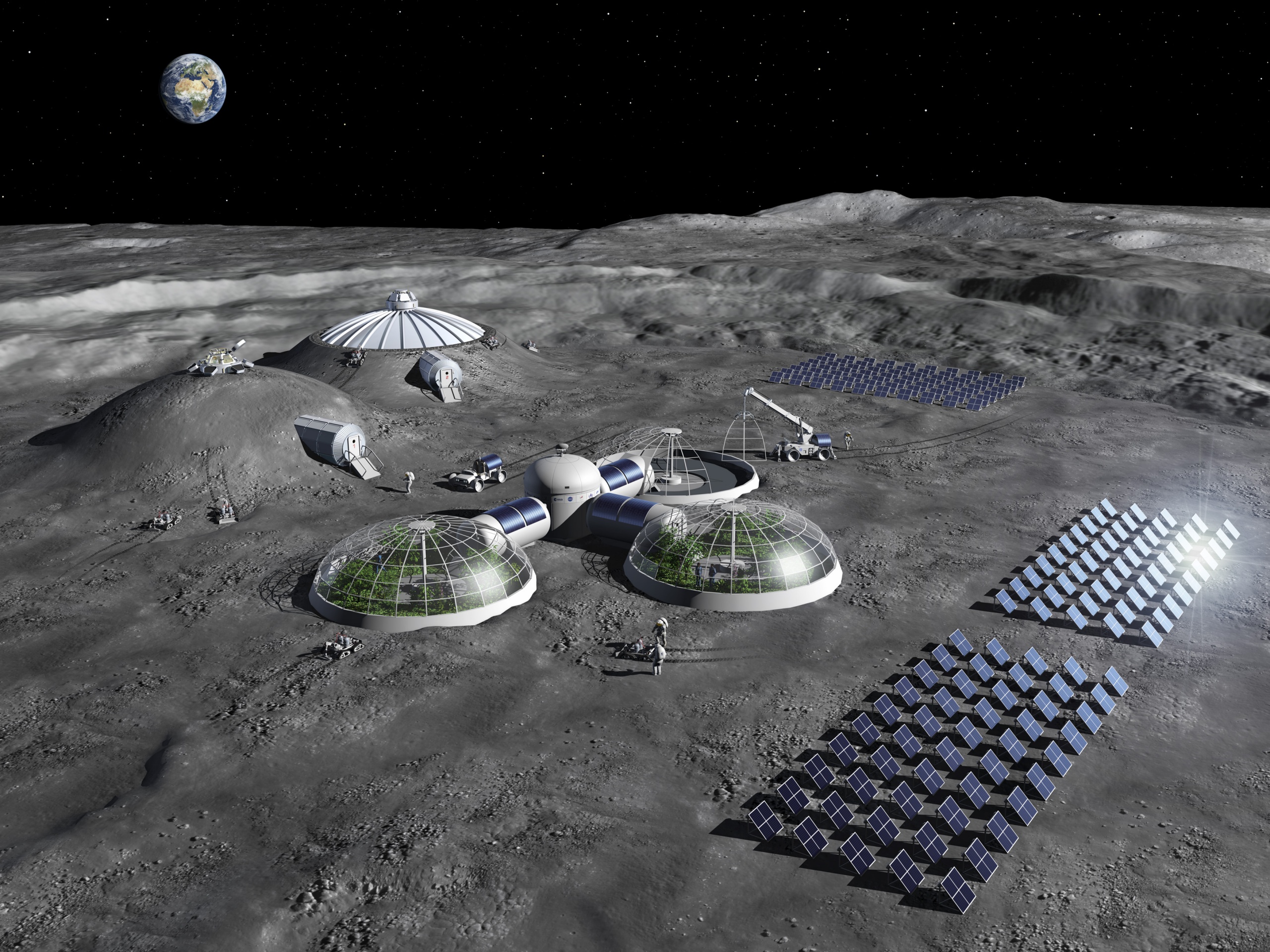
First up, a consortium of engineers presented data that shows just how water and oxygen can be extracted from lunar soil, one of the objectives to support future bases on the Moon. Per their press release, the process works like this: In the experimental set-up, the soil simulant is vaporized in the presence of hydrogen and methane, then “washed” with hydrogen gas. Heated by a furnace to temperatures of around 1000 degrees Celsius, the minerals turn directly from a solid to a gas, missing out a molten phase, which reduces the complexity of the technology needed. Gases produced and residual methane are sent to a catalytic converter and a condenser that separates out water. Oxygen can then be extracted through electrolysis. By-products of methane and hydrogen are recycled in the system.
Prof Michèle Lavagna, who led the experiments, notes: Our experiments show that the rig is scalable and can operate in an almost completely self-sustained closed loop, without the need for human intervention and without getting clogged up.
Congrats to the team on moving forward with in situ resource management.
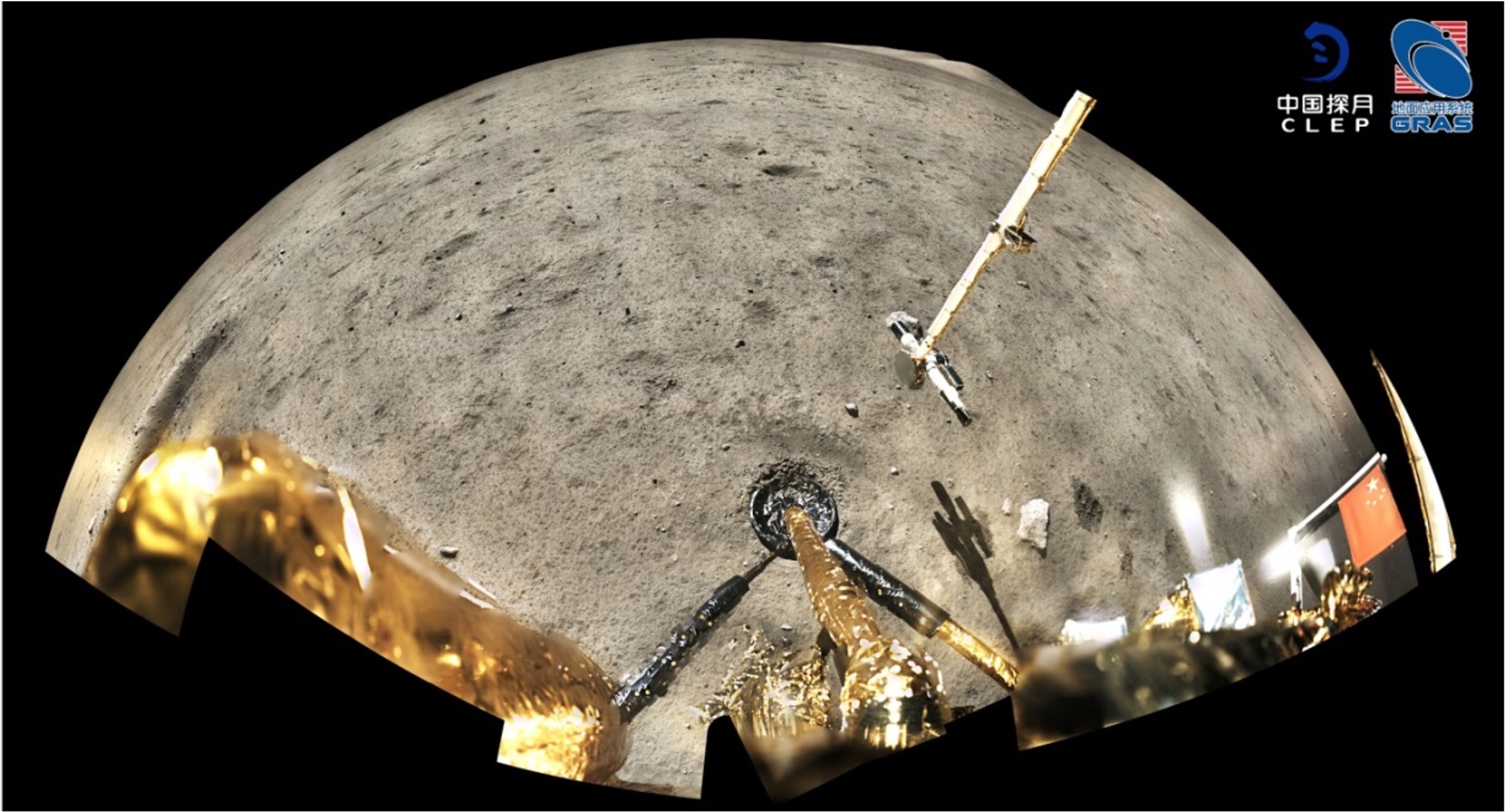
Speaking of Moon rocks, near the end of last year… or rather 2020… which was only last year, right? Anyway, near the end of 2020, China’s Chang’e-5 mission returned sampled rocks from the lunar surface to Earth. About two kilograms of material was collected, and an initial analysis was presented at the conference by graduate student Yuqi Qian.
Those initial results show that nearly 90% of the recovered sample was from the landing site in the Northern Oceanus Procellarum and were mare basalts, those darker grey areas we see on the Moon as opposed to the lighter grey highland material. However, the remaining ten percent of the sample is considered “exotic” because the fragments seem to come from either other places on the Moon or space rocks or both.
Per the press release: The team has modeled the potential contributions from specific craters to the south and southeast (Aristarchus, Kepler, and Copernicus), northwest (Harding), and northeast (Harpalus). Qian’s findings show that Harpalus is a significant contributor of many exotic fragments among Chang’e-5’s sample haul… Some fragments may have been thrown into [the] Chang’e-5 landing area from nearly 1,300 kilometers away.
We’re definitely looking forward to more results from this sample.
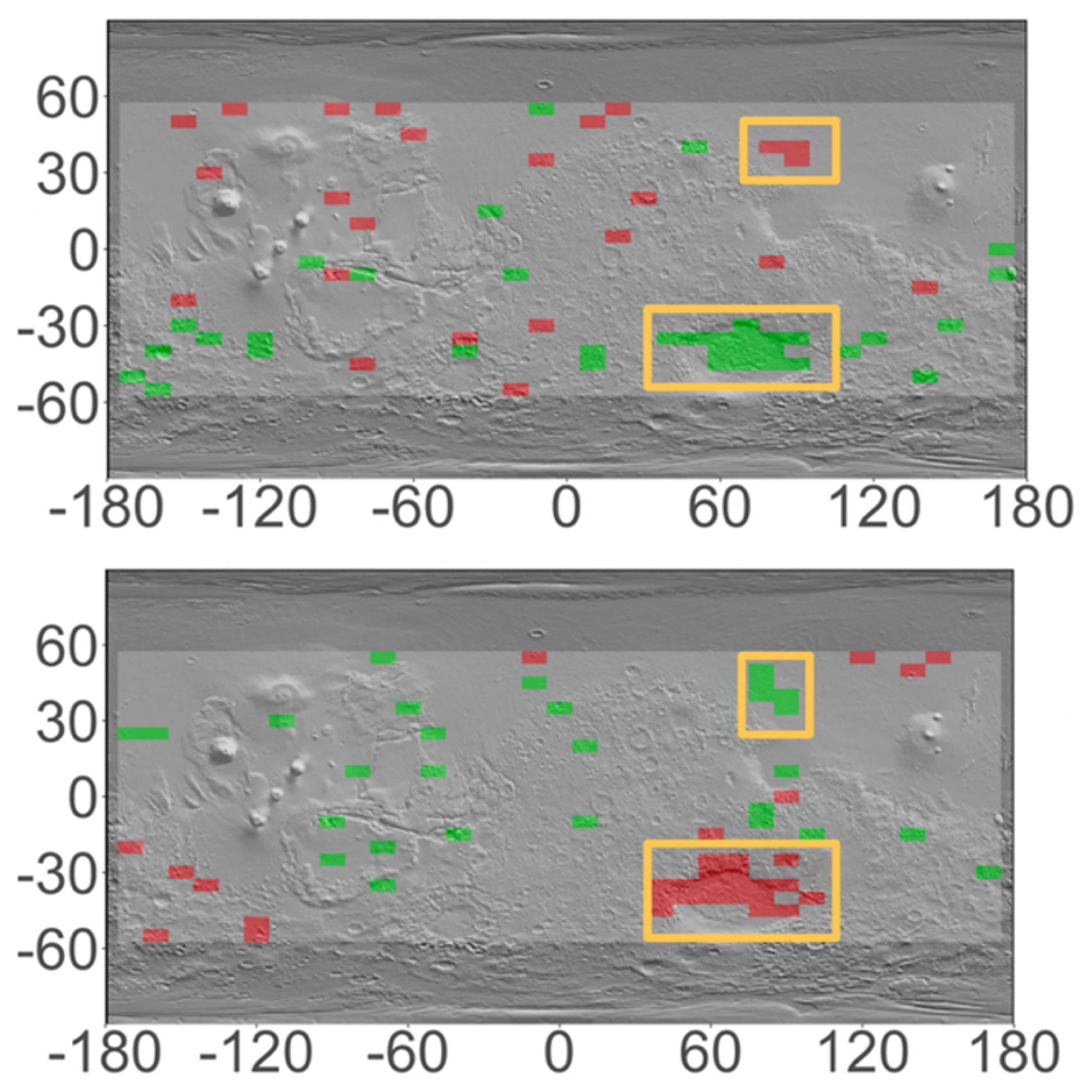
Finally, in our last story from EPSC, we move farther out to Mars and a presentation on how a team of scientists has used seasonal variations in the levels of hydrogen to identify possible sub-surface water ice deposits. The data collected comes from NASA’s Mars Odyssey spacecraft, which has been orbiting Mars for nearly twenty years. And two regions specifically were studied as areas of interest: Hellas Planitia and Utopia Rupes in the southern and northern hemispheres, respectively.
Presenter Dr. Germán Martínez explains: Data from Mars Odyssey’s Neutron Spectrometer showed signs of hydrogen beneath the surface of Mars from mid to equatorial latitudes, but we still had the challenge of working out whether this is in the form of water ice, which can readily be used as a resource or locked away in mineral salts or in soil grains and minerals. This is where the seasonal variation provides an important clue. As the coldest ground temperatures occur at the same time as the largest observed increase in hydrogen content, it suggests that water ice is forming in the shallow subsurface of these regions during the fall and winter seasons, and then sublimating into gas during the warm season of each hemisphere.
Finding water ice in the mid-latitudes is incredibly important for future crewed missions to Mars. We know there is water ice at the poles, but the extreme cold and lack of solar light limits just how easy it would be to access that water ice. To truly be useful to humans on Mars, we’ll need to find resources we can access, and it seems that this study has given us two more areas to look at as possibilities.
Martínez notes that the plan going forward is to continue studying the mineralogy of the two regions using different instruments to see if they can find rocks that have been altered by water. Perhaps they will have those results for us at next year’s conference.
When we think of our planet Earth, we like to think of it as a solid object that we can’t really mess up. Unfortunately, the Earth is neither solid nor too large for us humans to be able to change.
Fun fact: When the massive Three Gorges Dam in China was filled with water, it actually slowed the Earth’s rotation down by changing the planet’s distribution of mass. The change was only a fraction of a microsecond, but every time we change the distribution of water on the planet’s surface, we change how the planet rotates. We also change how the crust of the planet beneath that water is shaped.
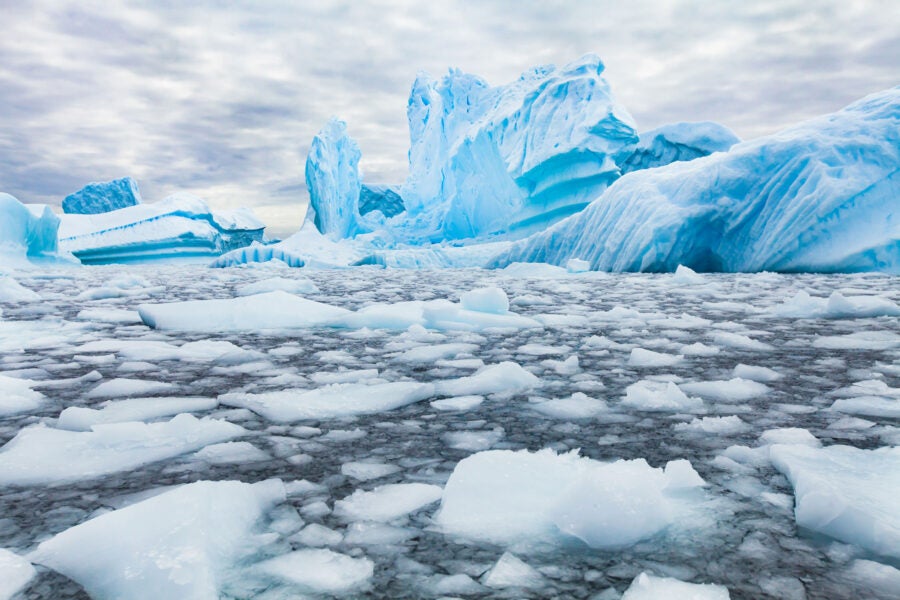
And here is your regular reminder that water comes in many forms, including ice and snow that can build up over centuries and melt away over years.
Here’s another fun fact: Volcanoes in icy climates erupt more often after the spring thaw.
It turns out the weight of snow and ice on the crust of our world is enough to shape our planet, like heavy books set on a memory foam mattress. And today, with that snow and ice and oh-so-many glaciers melting, the weight of the world is being redistributed.
According to a new paper in Geophysical Research Letters and led by Sophie Coulson, this melting is causing changes in the 3-D shape of the Earth’s crust to an extent that we hadn’t anticipated. Some of you may be old enough to remember the old mattress ads that showed bowling balls being dropped on mattresses next to glasses of drink. With the good mattresses, the ball’s motion would be absorbed and the glass would stand still. With the bad mattresses, the entire surface would deform, and the glass would spill.
It turns out the Earth is like a bad mattress, and the crust rebounds over huge distances with the removal of glaciers and the ice and snow around them. Understanding exactly what is going on is complicated by geologic time. It takes millennia for the Earth to return to its default shape, and we still can sense the rebound of the landscapes that were crushed under the last ice age, which lasted from 2.5 million to 11,000 years ago.
In this research, Coulson and her collaborators had to untangle motions from past melting and modern melting to understand what today’s global warming is doing to the shape of our planet, and the answer is: it’s doing a lot. The changes in shape that are being seen are over vast distances and are having effects on future melting.
Specifically, as the ground rebounds from the weight of now melted ice, it is tilting the landscape and making it hard to predict how the remaining ice’s motion and melting may be altered. Imagine seeing a mattress with a sleeper surrounded by bowling balls, but you aren’t sure if it’s a lumpy mattress or a smooth mattress. It might turn out that if you remove the bowling balls the rebounding mattress will leave behind lumps that send that sleeper sliding to a new position. We just can’t know. And with the Earth, thanks to Coulson, we now know one more thing we don’t fully know, and the list of unknown unknowns has gotten just a little bit smaller.
Meanwhile, in the world of planetary formation, it feels like every week brings new unknowns as often as solved mysteries.
Let’s lay out some of the basics. In your mind’s eye, picture a newly formed star with a disk of gas and dust orbiting around it. Soon, that disk begins to have gaps, as small protoplanets begin to clump together, clearing paths as they orbit. Then the increasing gravity of those clumps pulls in more and more dust and gas until a planet actually exists in a lovely cleared orbit.
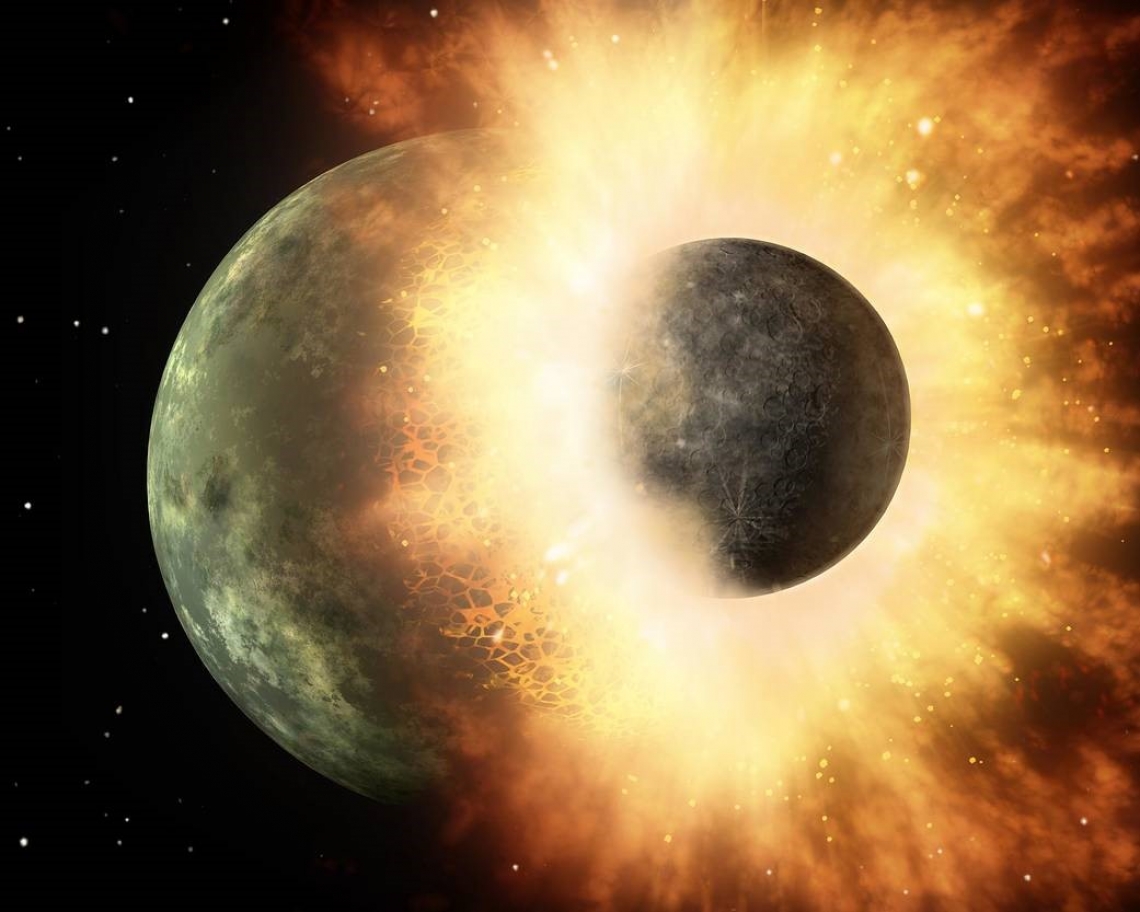
Yes, that’s the basic idea. Basics, however, never seem to satisfy scientists, who want to understand every detail possible and how changes in the steps affect the entire process. So research led by the University of Arizona Lunar and Planetary Laboratory set out to understand just how the conventional view of planetary accretion, where those tiny baby planets get bigger and bigger, exactly works. And, of course, it probably doesn’t work quite the way we thought. Quel surprise.
Rather than gently rounding up material as they rotate and revolve, it now seems that growing planets are more like billiard balls, running amok in their orbits and colliding again and again with each other until their momentum is slowed down enough for a collision to stick.
Computer models are amazing, y’all.
Lead author Erik Ashpaug explains: We find that most giant impacts, even relatively ‘slow’ ones, are hit-and-runs. This means that for two planets to merge, you usually first have to slow them down in a hit-and-run collision. To think of giant impacts, for instance, the formation of the Moon, as a singular event is probably wrong. More likely it took two collisions in a row.
Okay, so not only is our concept of a gently-ish building planet wrong but so is our current hypothesis on the formation of the Moon? That’s partially true. Yes, Earth likely got hit by a Mars-sized body we refer to as Theia, but instead of kicking out a ton of Earth’s material on the first pass, Theia skimmed the newly forming planet instead, losing momentum. About a million years later, Theia returned, hit the Earth again, and this time was slow enough to partially stick and partially remove a bit of Earth to create a satellite with the same isotopic chemistry as the parent body. Asphaug notes: The double impact mixes things up much more than a single event, which could explain the isotopic similarity of Earth and moon, and also how the second, slow, merging collision would have happened in the first place.
On top of this new hypothesis, one of the two papers published in The Planetary Science Journal proposes that Earth provided a bit of a vanguard for Venus, which means that when objects hit Earth, they lost energy and continued closer to the Sun. They would then, at this lower energy level, collide with Venus and stick. That’s a result of the Sun’s gravity well, which is strongest closer to the Sun, so things that move in and slow down aren’t going to come back out of that well. They no longer have the energy to escape, and they’re low-energy enough that a collision means Venus gets to grow.
But what about Mercury and Mars? They are probably what’s left of that earliest population of baby planets that just never got hit all that often because Earth pulled the other lumps in and slowed them down, and then Venus made them stick. No lumps for you, Mercury!
Of course, as always, this research has led to more questions. How did Earth grow as big as Venus? Why is Earth’s magnetic field so much stronger than Venus? Why doesn’t Venus have a moon if it was hit in a similar fashion to Earth?
Planetary formation. Causing me headaches for years now. We’ll keep bringing you these stories, but for now, it seems that the mystery of planetary formation got a few more unknowns.
Who knew that a story about a black hole consuming a star would be the most chipper news of the day? But hey, it is!
At some point not too long ago, on cosmic time scales at least, one star in a large star cluster had a really bad day. At a certain point in its orbit through the cluster, it strayed too close to a black hole and began to be disrupted. As it spiraled closer, it was messily torn apart more and more until it wasn’t so much a star as a cloud of hot, excited debris and the radiation given off just as some of that shredded star fell into the black hole. Thanks to the simple fact that the black hole shredded the star instead of devouring it all at once, scientists were left with a unique chance to use that spiraling debris to measure the mass of that black hole.
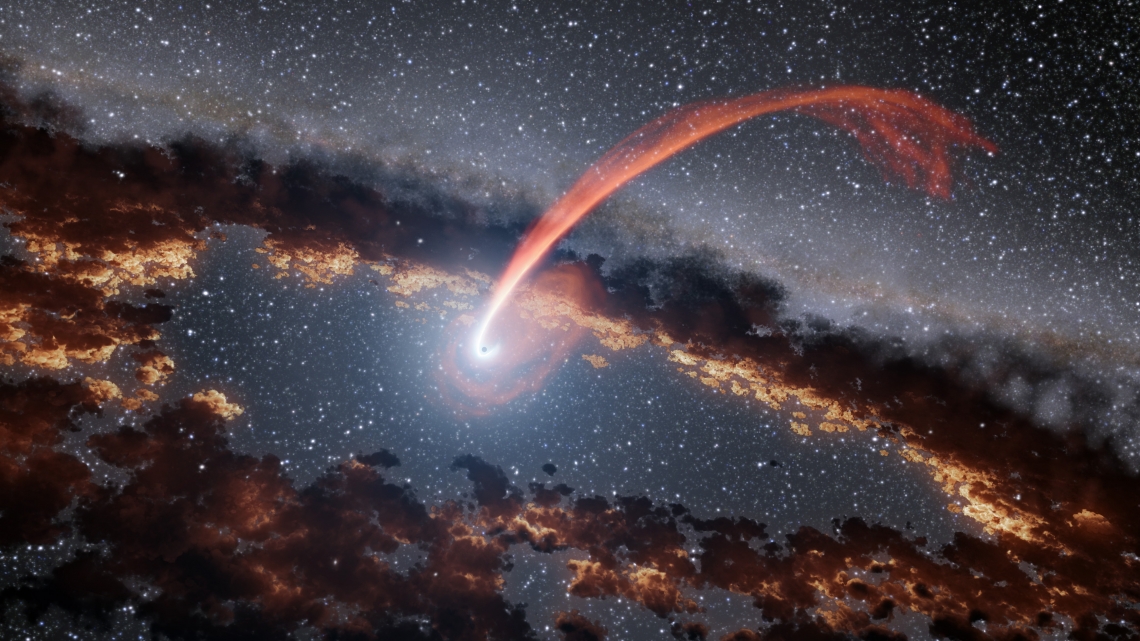
This isn’t a straightforward process. When a planet orbits a star, the bulk of the mass is in the star, and the planet’s motion is easy to see separate from the star’s motion. The rotation rates of the star and planet really have close to nothing to do with how the objects move. With this shredded star and invisible black hole, things are a lot more complicated.
First off, that black hole can’t be directly observed. And instead of a single moving object, there is a spinning disk that doesn’t all spin together. And thanks to relativity, that disk’s motion does not reflect normal Newtonian orbital dynamics and a lot of relativity. This system is trying really hard to hide its secrets. That said, a team of researchers led by Sixeng Wen has published a paper in The Astrophysical Journal that uses that swirling debris disk and a whole lot of math to calculate both the spin and mass of the black hole.
And that mass is pretty interesting. This black hole in a star cluster appears to be intermediate in size, between the mass of black holes that form directly from collapsing stars and supermassive black holes in the centers of galaxies that can be millions of solar masses in size.
How the universe created supermassive black holes is a bit of a mystery, and one of the possible solutions is the merger of stellar-mass black holes until they are intermediate-mass, and this object in a nice dense star cluster where mergers statistically should happen is fifteen to twenty thousand solar masses, making it totally intermediate and exactly what we’re looking for.
And this system, which is only visible because it mostly ate a star and left remains behind for us to see, hints at the possibility that star clusters are full of intermediate-mass black holes – ones that are generally invisible because they haven’t been messy eaters.
While I’m not sure that a universe full of invisible black holes is an existential win, it is a scientifically super cool thought. And it is the thought we’re going to leave you with today.
This has been the Daily Space.
You can find more information on all our stories, including images, at DailySpace.org. As always, we’re here thanks to the donations of people like you. If you like our content, please consider joining our Patreon at Patreon.com/CosmoQuestX.
Credits
Written by Pamela Gay and Beth Johnson
Hosted by Pamela Gay and Beth Johnson
Audio and Video Editing by Ally Pelphrey
Content Editing by Beth Johnson
Intro and Outro music by Kevin MacLeod, https://incompetech.com/music/