Researchers looked for a slowdown in black hole rotational speeds due to the collection of ultralight bosons, but they found nothing, eliminating the hypothetical particle from the list of possible dark matter particles. Plus, neutrino hunting, neutron stars, a space hurricane, and our review of some delightfully nerdy apparel.
Media
Transcript
Hello and welcome to the Daily Space. I am your host Dr. Pamela Gay.
And I am your host Beth Johnson.
And we are here to put science in your brain.
While we love to tell you what we know, let’s face it, sometimes it’s much more interesting to work through a “whodunnit?” mystery involving other galaxies than to try and make sense of any more terrestrial mysteries.
In our first story of the day, we have the mystery of weird chemical ratios in a dwarf galaxy 430 million light-years away in the direction of the Hercules constellation. This little system is a leftover bit from the early universe that, like a bit of an unmixed splattered recipe, doesn’t contain all the ingredients or even the existing ingredients mixed as expected.
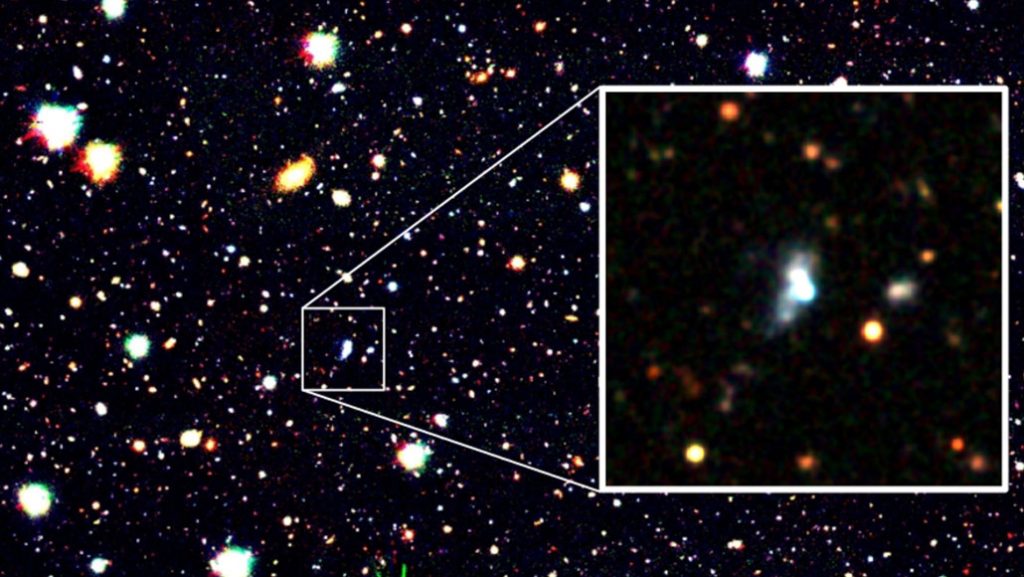
This particular system is mostly made of the base hydrogen and helium our universe formed with, and it is enhanced by a touch of oxygen that was created in massive stars. Surprisingly, these systems also have an unexpectedly large dash of iron. Normally, we expect to see an iron-to-oxygen ratio that reflects the kinds of stars that create these elements under normal conditions. Iron comes from long-lived stars that explode as supernovae, and oxygen comes from smaller stars that exhale their materials into the space around them. The rarity of the supernova leads to typically less iron than oxygen, but that’s not what we see in this weird galaxy, and this is the second galaxy like this we’ve found.
To explain these two galaxies, astronomers considered the differences between stars made today and made in the early universe and found that massive stars made prior to the creation of heavy elements could have formed both iron and oxygen, creating the chemical recipe we see in these systems. Because these galaxies are small, once those massive stars formed, nothing was really left to make more massive stars. If this theory is true, these systems should contain intermediate-sized black holes – black holes a hundred times the mass of the Sun.
It’s unclear how we might observe such black holes in these systems, but it is still cool that we have a theory for what happened that has an observational test. And maybe someday we’ll find a way to see the effects of those possible black holes.
Trying to figure out how to observe things takes up a shocking amount of time. Sure, you can go out, look up, and do some science – especially variable star science – with your eyeballs. It turns out that, for every easy thing to observe, there are a dozen things trying not to be observed. Things like neutrinos.
These antisocial particles are generated in a lot of different nuclear processes, ranging from fission to fusion to everyday radioactive decay, and high-speed collisions. These particles generally pass through us by the thousands without anything happening, but occasionally, a neutrino with just the right energy on just the right path can collide with a particle in water and give off a flash of light.
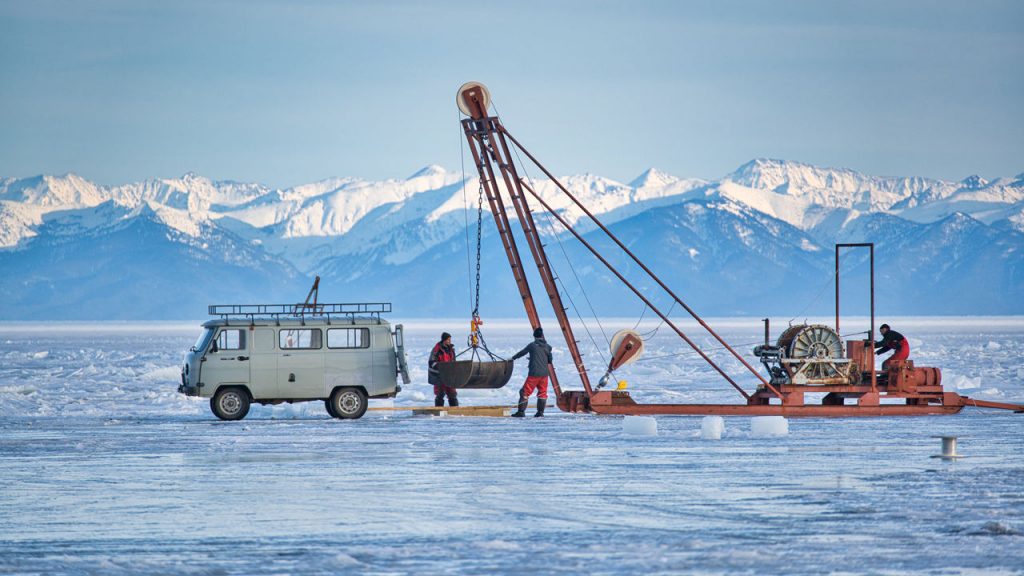
Our human bodies are far too small for this to be a concern, but scientists can leverage giant regions of water or ice to look for these flashes, and right now, there are new detectors being built by stringing sensors in grids in the Mediterranean, in Russia’s Lake Baikal, and off the western coast of Canada. With this global network of detectors, it’s hoped that it will become possible to figure out what kinds of phenomena are producing neutrinos.
Currently, a massive detector named IceCube is looking for flashes in Antarctic ice. Two of these neutrinos are traced back to events associated with supermassive black holes in galaxy cores. Since we thought these neutrinos came from star-forming regions and supernovae, these discoveries highlight the need for more information. Now, thanks to the Earth having so much water and the ability of scientists to string sensors together and dangle them in that water, the detailed information we want should be coming.
Finding neutrinos is hard, and not all neutrinos are identical. We know there are neutrinos related to reactions with electrons, muons, and tau particles, and each of these sibling neutrinos has its own mass and its own slightly different way of being found. These three flavors of neutrino are all part of the Standard Model and have all been observed, and they have been observed to oscillate from one kind to another as neutrinos exchange their mass for energy or their energy for mass.
In addition to these known particles, there may also be what are called sterile neutrinos or inert neutrinos that come in a wide range of masses that are waiting to be observed. As the theory goes, the known neutrinos have a particle spin that is called left-handed. Basically, if your thumb is in the direction of motion, the property called helicities, which is like a spin but not quite the same, is aligned like your fingers curving around the direction of motion. There is no reason, however, that the other orientation shouldn’t exist, that particles shouldn’t act like your right thumb is the velocity and your fingers are the helicities.
This “why aren’t they also flipped” question has led to the idea, to the hope that these alternate neutrinos, these sterile neutrinos, could be out there, refusing to interact with anything other than gravity and having masses anywhere from 1 eV to 10^15 GeV. And there could be many kinds of them with many different masses that together add up to explain dark matter. Trying to discover particles that don’t want to interact except via gravity, however, is a challenge.
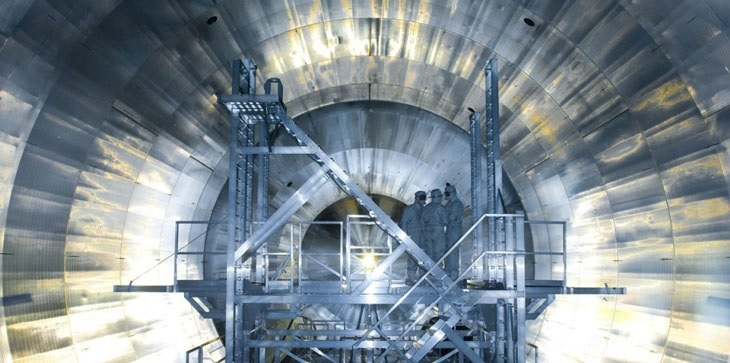
Luckily the KATRIN experiment has accepted this challenge, and in a new paper in Physical Review Letters, they describe how they are looking for sterile neutrinos alongside known neutrinos. Basically, in heavy water, protons will periodically be converted into neutrons, and give off one electron and one neutrino in the process. The entire system has a decay energy of 18.6 keV that can spread between their motions, and the neutrino’s energy can get measured as the flashes created when it hits something, and thanks to probabilities, we’ll see a range of energies in those flashes that reflect how often those neutrinos get how much of that 18.6 keV. If sterile neutrinos are formed, we should see a different family of flashes that reflect the energy of the sterile neutrinos.
So far, the KATRIN experiment has been able to rule out masses between 3 and 30 eV for sterile neutrinos. If sterile neutrinos are out there, they are probably kinda big, and this is what we need to explain dark matter. The idea that dark matter is just a flipped version of something we already know about is one that really appeals to me. It doesn’t wreck the Standard Model but expands it in a sensible direction. Hopefully, KATRIN will stop ruling out masses for sterile neutrinos and start finding sterile neutrinos.
For now, though, we wait and wonder what is dark matter and what particles are out there, outside the incomplete Standard Model, waiting to be found.
We say the Standard Model is incomplete because we know the universe’s mass-energy allotment consists of mostly dark energy, then a lot of dark matter that gravitationally throws its mass around while refusing to be seen, and finally a tiny amount of regular material like we’re made of. The Standard Model of Particle Physics does a good job explaining all that visible, detectible stuff we call baryonic matter. But it offers no solutions for dark matter.
The Standard Model is also based on observations and realizing that if we see X and Y, there is probably also a Z, but not having solid physics to explain why X and Y and Z were ever needed in the first place. As we’ve said before, this is like the point when Kepler could explain planetary positions with his three laws of orbital motion, but he didn’t have gravity to explain why the equations worked. The theory was good; it was just missing the underlying physics. Particle physics is currently at that stage. The Standard Model is good, but we know it is missing things.
Dark matter – 27% of the makeup of the universe – it’s a missing thing.
And because it only interacts with gravity (or when it randomly collides with something), scientists are looking at every collision and every gravity field for signs of dark matter.
In addition to thinking dark matter could be sterile neutrinos, particle physicists think a hypothetical particle called an ultralight boson is also a possibility. In general, bosons are particles that carry force or other properties. The Higgs boson carries mass. The photon carries the electromagnetic force. Gluons and W and Z particles carry the strong and weak forces. It’s unclear what ultralight bosons might carry, but if they are out there, they should be interacting. One possible kind of ultralight boson is the axion, and if it is out there, it should be interacting with spinning black holes.
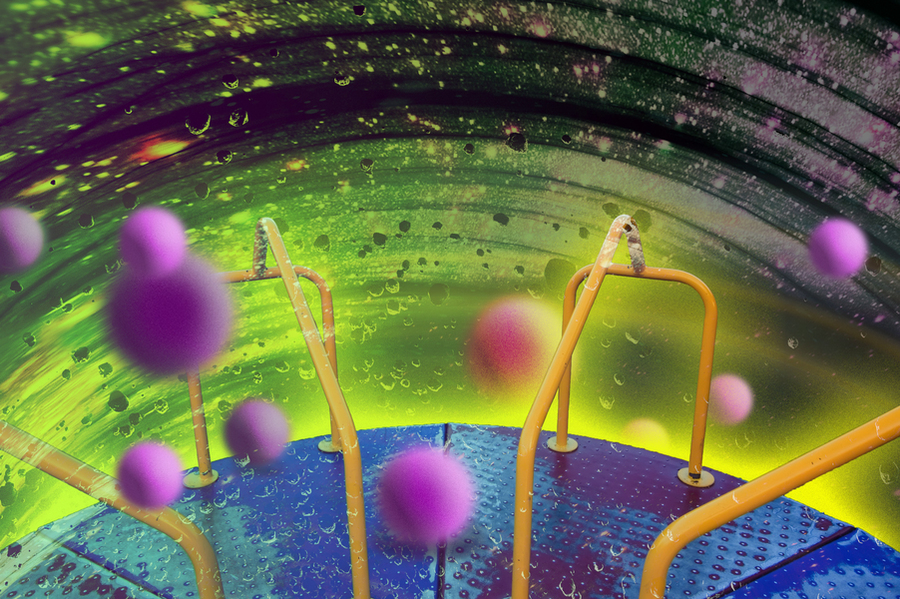
As the theory goes, a supermassive black hole should pull in clouds of ultralight bosons because let’s face it, they’ll pull in anything that gets too close. Since the bosons don’t have the same motion as the spinning black hole, when their slow-motion selves get pulled in, the black hole’s spin should slow. This is like what happens when someone hops onto a spinning playground carousel: its rotation spins as it gains their not-moving-fast-enough mass.
In looking at supermassive black holes, scientists have realized that fast-rotating systems put limits on what mass the theoretical ultralight bosons might have. If we find fast-spinning, old black holes, that means there probably aren’t a large mass of ultralight bosons out there slowing things down as they hop on or off the spinning black hole like kids on a carousel.
And looking at the black holes GW190412 and GW190517, scientists have determined these two old supermassive black holes are rotating pretty much as fast as a supermassive black hole can rotate, which is twice as fast as would be expected if ultralight bosons exist. This means that ultralight bosons, if they are out there, aren’t the ultralight masses we imagined. They could exist just at an even smaller mass than imagined, but…
The more we look, the more we aren’t finding things, and it is starting to get kind of annoying. But this is why scientists do science; we want to figure out these problems with the universe, and some problems are just going to take us a while to sort.
Now let’s solve a terrestrial mystery after all.
Aurorae are nothing new. We’ve talked about them before, whether it was citizen scientists helping understand the STEVE phenomenon or aurorae on other planets like Jupiter and Saturn, we are all fascinated by their beauty. And yet, there are still unexplained phenomena when it comes to aurorae. Today, however, there is one fewer mystery. Allow me to introduce you to the space hurricane.
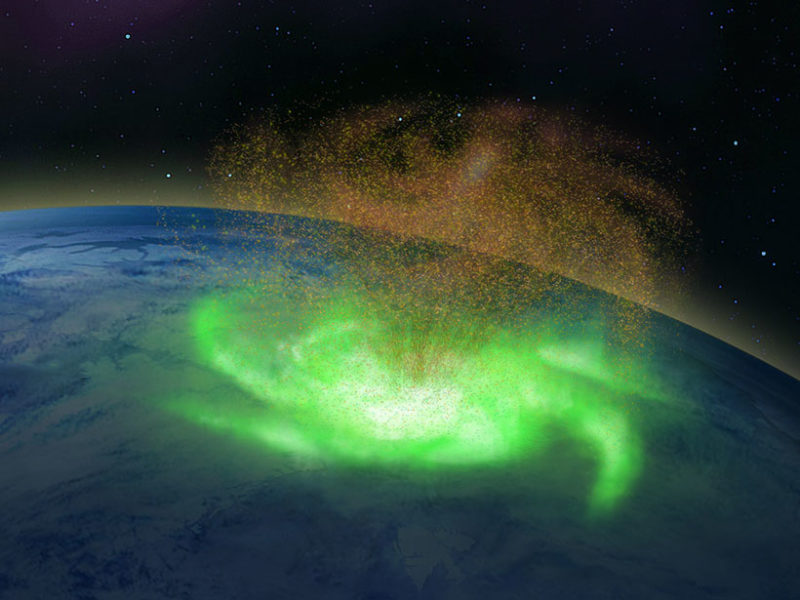
Let’s start with the basics. Aurorae occur when charged particles, electrons generally, interact with atoms high in the Earth’s atmosphere. The electrons change the state of the atoms, increasing their energy, but that isn’t a stable state. The atoms then lose that energy and return to their original stable state, and the energy is released as photons of light, creating the gorgeous colors in the sky.
Where do the charged particles come from? The Sun. In particular, they are carried on the solar wind, and when the Sun is in its more active phases, we frequently get aurora alerts. Anytime there is a coronal mass ejection, we can definitely expect aurorae in the highest latitudes around the polar regions. If an ejection is particularly large, the aurora can extend into the mid-latitudes. I know my family in Minnesota has been able to see them.
However, aurorae don’t just happen when the Sun is energetic. There have been numerous instances of spectacular aurorae occurring when the Sun is quiet and there is little solar wind. So a research team combed through thousands of aurora images to understand why these events occurred. They found several dozen instances, and one event, in particular, lasted for eight hours, giving the team a lot of data to work with. And what they found was that these aurorae were spiral-shaped and circular like a hurricane.
That eight-hour hurricane occurred on August 20, 2014, and it was made of spiral arms of plasma that were over 965 kilometers long, spinning around a calm center. Instead of water falling, these atmospheric storms rained electrons over the magnetic North Pole.
Unlike their more terrestrial counterparts, these space hurricanes aren’t a large risk to humans on the surface, but they are important to understand because all that energy can cause fluctuations in radio waves that pass through the ionosphere. That could affect our satellite communication and maybe even navigation. Plus these storms might heat up the upper atmosphere, which would expand as a result, changing in density, and all of that becomes an issue for the satellites and space debris that orbit at that elevation. Change the drag on a craft, and you change its velocity. That makes orbital predictions more difficult.
As coauthor Larry Lyons notes: If you want to know where the space station is going to be a few hours from now, you have to know what kind of an atmosphere it’s going through.
There are still a lot of open questions about these newly discovered space hurricanes, and lead author Qing-He Zhang lays them out: What controls the rotation of space hurricanes? Are these space storms seasonal like their tropical counterparts, perhaps limited to the summer when the Earth’s magnetic dipole is tilted just the right way? And can space hurricanes be forecasted like weather events on Earth?
Pamela and I are both excited to see where this new avenue of research goes. Aurorae are just cool.
This has been the Daily Space.
Learn More
Oxygen-poor Galaxy May Contain Stellar Shrapnel
- Science News article
- “EMPRESS. II. Highly Fe-Enriched Metal-poor Galaxies with ∼1.0 (Fe/O)⊙ and 0.02 (O/H)⊙ : Possible Traces of Super Massive (>300M⊙) Stars in Early Galaxies,” Takashi Kojima et al., to be published in The Astrophysical Journal (preprint on arxiv.org)
The Underwater Search For Neutrinos
- Science article
KATRIN Experiment Hunts For Sterile Neutrinos
- Max Planck Institute for Physics press release
- “Bound on 3+1 Active-Sterile Neutrino Mixing from the First Four-Week Science Run of KATRIN,” M. Aker et al, 2021 March 5, Physical Review Letters
Ultralight Bosons Not Found Around Fast-Spinning Black Holes
- MIT press release
- “Constraints on Ultralight Scalar Bosons within Black Hole Spin Measurements from the LIGO-Virgo GWTC-2,” Ken K. Y. Ng, Salvatore Vitale, Otto A. Hannuksela, and Tjonnie G. F. Li, 2021 April 14, Physical Review Letters
New Aurora Phenomenon Identified: The Space Hurricane
- Eos article
- “A space hurricane over the Earth’s polar ionosphere,” Qing-He Zhang et al., 2021 February 22, Nature Communications
Credits
Written by Pamela Gay and Beth Johnson
Hosted by Pamela Gay and Beth Johnson
Audio and Video Editing by Ally Pelphrey
Content Editing by Beth Johnson
Intro and Outro music by Kevin MacLeod, https://incompetech.com/music/