New research looking to explain the “missing” portion of baryonic matter in the universe managed to locate the material lurking in intergalactic space as hot, low-density gas. Plus, supercontinents, Enceladus, and weird earthquakes.
Media
Transcript
Hello and welcome to the Daily Space. I am your host Dr. Pamela Gay.
And I am here to put science in your brain.
Next week, the CosmoQuest staff is taking the week off as people on our team celebrate Passover and Easter, take a spring break, and in my case, just take the time to gather all the misplaced coffee cups that have accumulated in strange corners since we last took a week off. Today, I’ll be covering the news solo, as Beth has taken off early.
The news, however, keeps coming. And our first story of the day is the kind of science that once I would have said is impossible.
With light, there is no way to see what was happening in the universe prior to the release of the Cosmic Microwave Background (CMB). Prior to that moment, the universe was opaque and completely hidden to our telescopes. If you asked me if we could ever observationally know anything about the universe’s first 400,000 years, I would have sighed and said I don’t think so; that time is hidden except for the echoes it left imprinted on the CMB.
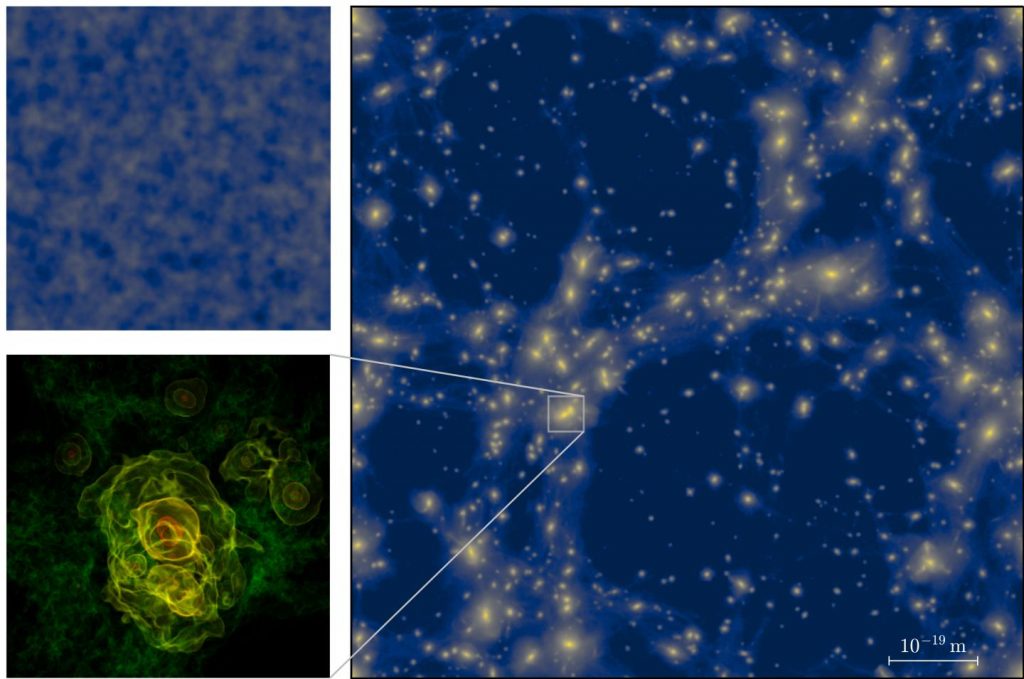
What I hadn’t counted on was gravitational wave science advancing the way it has, with people not just using detectors like LIGO and Virgo to measure black hole mergers, but also teams using the timing of pulsars to detect the background perturbations in space caused by events that occurred early in the universe, before that CMB was released. Through gravitational waves, we can now imagine testing theories about what happened in the earliest moments of this universe.
Researchers at the universities of Gottingen and Auckland created a simulation to look at the evolving structure of the universe in its first seconds. They were able to see a complex network of structures similar in form to today’s large-scale structure of the universe, only much, much smaller. According to Ph.D. student and lead author of this study, Benedikt Eggemeier: The formation of such structures, as well as their movements and interactions, must have generated a background noise of gravitational waves. With the help of our simulations, we can calculate the strength of this gravitational wave signal, which might be measurable in the future.
This is my favorite kind of theory paper. It is one that makes concrete predictions that can one day (maybe) be observed. What gets me about this work is the scale they were working at. The early universe was tiny. As Jens Neimeyer explains it: The physical space represented by our simulation would fit into a single proton a million times over. It is probably the largest simulation of the smallest area of the Universe that has been carried out so far.
Here is to hoping we one day learn how to record early gravitational waves with the same detail as the CMB.
Computer models are becoming more and more important to transforming all our measurements and ideas into things we can try and measure. This is as true for the universe as a whole as it is for our home planet, Earth. We can’t go back in time and see directly how the continents have moved and evolved over the planet’s five billion-year history. We can, however, find the world’s oldest rocks and use them to place limits on what might have happened in the past.
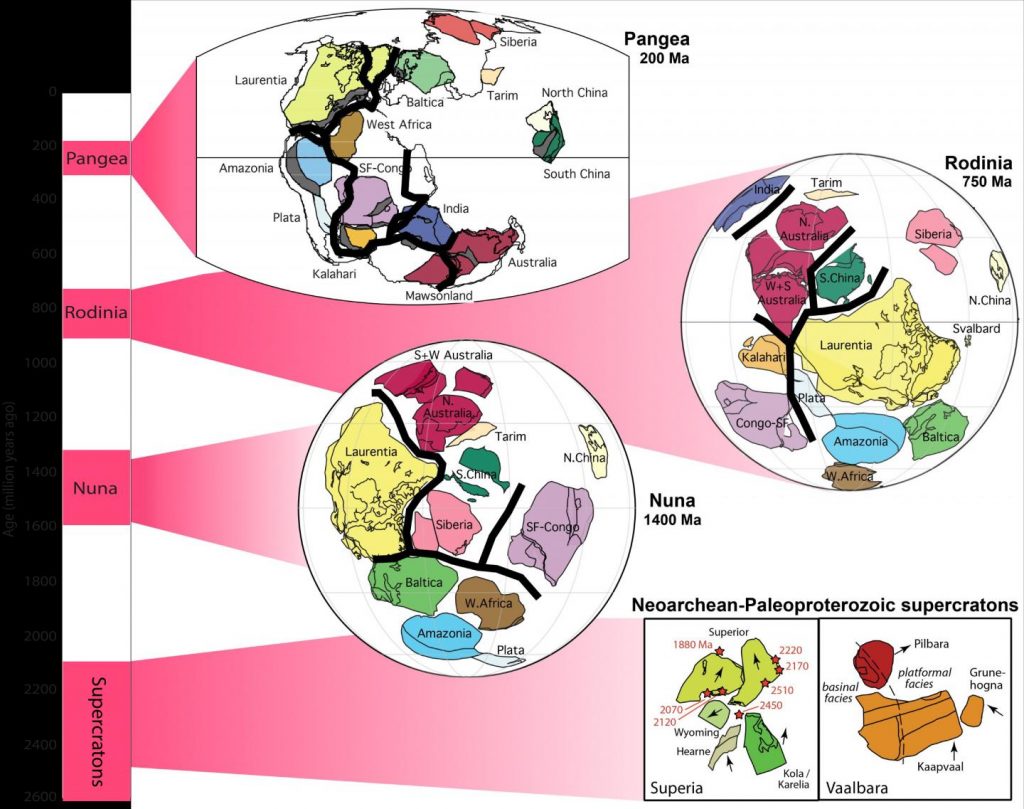
We’ve known for about a century that all the modern continents once fit together into a single supercontinent named Pangaea. More recent work has found evidence of two older supercontinents and a 600 million-year continental cycle. Has that always been the case? Without evidence, it wasn’t something a model alone could sort, so researchers found the oldest rocks out there, and went looking for magnetic evidence of how those rocks were once aligned with the Earth’s magnetic field.
Based on these new measurements and models of our world, this team concluded, to quote Zheng-Xiang Li: We can almost rule out the existence of a long-lived single supercontinent before two billion years ago (2 Ga), although transient supercontinents may have existed. More likely, there could have been two long-lived clusters of cratons, or supercratons, before 2 Ga that were geographically isolated from each other, never forming a singular supercontinent.
This work is published in the journal Geology, with first author Yebo Liu, who adds: This study surely isn’t the final word on the debate, but it’s certainly a step in the right direction and we need to collect data from a lot more similar rocks to further test the hypotheses.
This entwining of data and models is key. If a model doesn’t have enough data, it is likely to miss important details. When it comes to understanding worlds, chemistry can play a significant role that is easy to forget. The Cassini mission to Saturn was able to catch samples of material from the geysers on Enceladus and determined that the moon has salty sub-surface seas. Gravitational measurements, and heat calculations, in combination with these salty measurements, build up a picture of ice building up at the equator and melting at the poles, likely creating a gradient in salinity as we see in Earth’s southern seas.
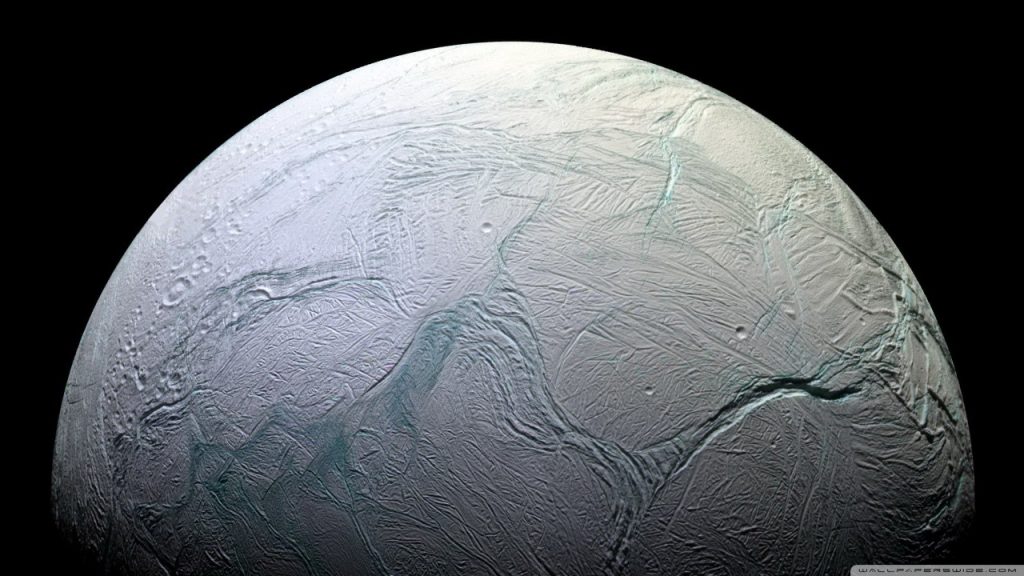
When saltwater freezes, the salt gets left behind in the unmelted water, making that water heavier, and driving currents. This work is published in Nature Geoscience with first author Ana Lobo who explains: Knowing the distribution of ice allows us to place constraints on circulation patterns.
Coauthor Andrew Thompson adds: Understanding which regions of the subsurface ocean might be the most hospitable to life as we know it could one day inform efforts to search for signs of life.
Data and models, working together, this is how we figure out the universe.
I love a good science mystery, especially when it involves earthquakes. Back in 2018, there was an earthquake in the region of Indonesia that caused a deadly tsunami, even though it wasn’t the usual type of fault system that causes tsunami. Scientists analyzed the quake and found that although the fault is technically a strike-slip fault, where two tectonic plates slide past each other, this fault was at a steep angle, so the sudden slippage of the two plates displaced enough water to set off the tsunami.
Now a pair of earthquakes in the Alaska-Aleutian subduction zone has given rise to a new mystery. You see, the first quake made sense. It happened as subduction quakes do, with one plate slipping below the other, and it happened in an area where quakes have happened before. A lot of happening going on here.
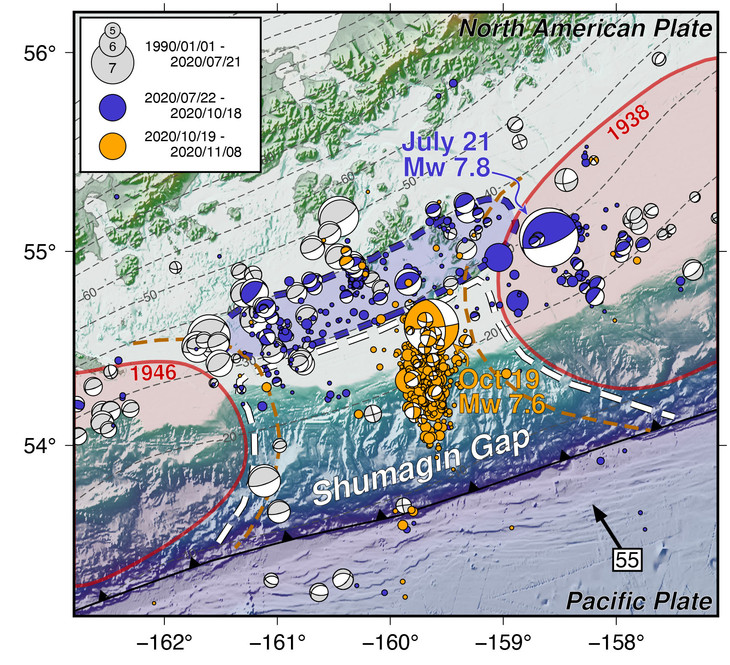
But the second quake. Well, that one didn’t happen as expected or where a quake would even be expected.
When it comes to earthquake fault zones, a lot of them have what we call a “gap” – a region along the zone where quakes do not tend to happen at all. It’s considered an unlocked or decoupled region. The rocks aren’t sticking together, so when the plates move, they move freely. No quaking going on. And this second quake in October 2020 not only occurred in a region of the subduction zone known as the Shumagin Gap, it was also a strike-slip earthquake, not a subduction quake.
A new paper analyzing these two earthquakes was published in Science Advances this week, and as lead author Kevin P. Furlong explained: There must be a fault in the subducting Pacific Plate, and we can’t see it. In the oceanic crust, there are strike-slip faults that develop at the mid-oceanic ridges. This fault in the Shumagin Gap could be a relic of a fault from the mid-ocean ridge, activated in a different way. It appears to be in the correct direction.
In more weirdness, that second quake also caused a small tsunami. Furlong and his co-author Matthew Herman modeled the two earthquakes along with the tsunami, and they found that the reason the second quake occurred is… well… because that section of the fault zone is unlocked. Herman noted: The potential for unusual earthquakes in these regions makes sense from our computational models. But it is still pretty counterintuitive that making the expected kind of earthquakes less likely actually makes other types of big earthquakes more likely.
So it turns out that we don’t completely understand all the nuances involved in plate tectonics. Earth is a big, complicated world, and we continue to analyze all the unusual events to dig at its secrets. Knowledge is powerful, and in this instance, knowledge could save the lives of people living in the region. The more you know and all that.
One of the great mysteries of the universe is what is dark matter. This bizarre material doesn’t interact with light but gravitationally throws light and mass around as it interacts with things at galaxy and galaxy cluster scales. People keep trying to identify dark matter with specific theorized particles and keep looking for new ways to make it go away with new observations. So far, dark matter refuses to be seen and refuses to go away.
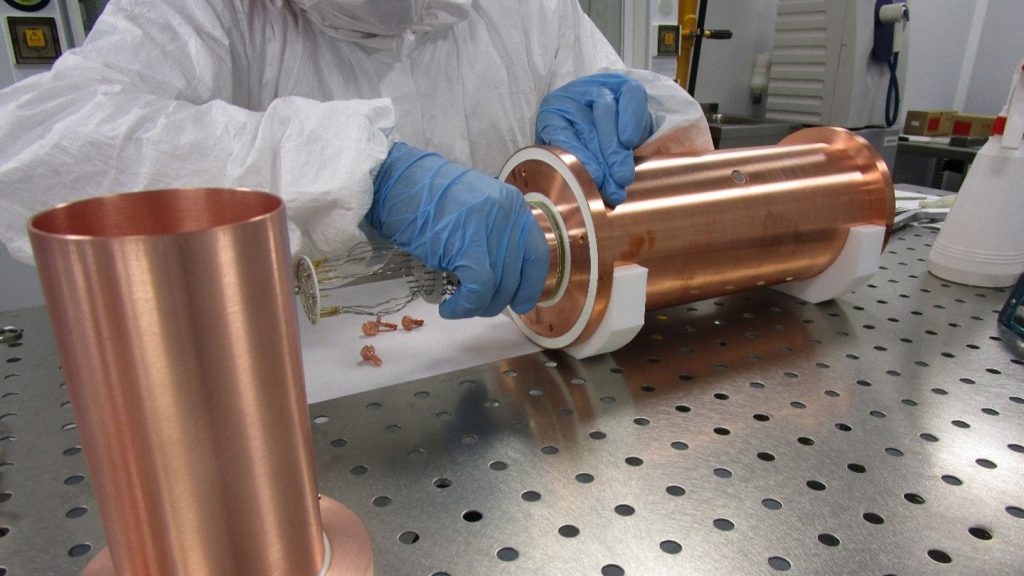
In the 1990s, there was some evidence that dark matter might be Weakly Interacting Massive Particles or WIMPs. This came from an Italian experiment that looked directly for WIMPs colliding with sodium iodide in a detector. It was expected that if WIMPs are dark matter, then they will be everywhere, and the detector would act as a windshield in rain, catching the WIMPs as we go through the galaxy. Specifically, it was expected that the Earth’s motion would seasonally change the rate at which WIMPs are detected as we move with and against the Sun’s motion around the Sun, just as the amount of rain hitting a windshield varies with velocity relative to the storm.
In 1998, the Italian team announced the detection of the expected signal, and in the twenty-plus years since, teams around the world have tried to find further evidence of WIMPs, to no avail. Most recently, a Spanish team – ANAIS collaboration – replicated the experiment in its entirety and found nothing, proving once again that to truly believe a result, you need to replicate that result. This seems to indicate WIMPs are not the dark matter particle we need.
This lack of detections brings into question more than just the possibility of dark matter being WIMPs. WIMPs are a key prediction of the supersymmetry model of particle physics. No WIMPs could mean no supersymmetry, which means that a whole lot of other predicted particles, for which we admittedly have no evidence, also don’t exist.
I personally am not bothered by this. The universe is what it is, and we’ll figure it out when we figure it out. There are, however, a lot of physicists who want there to be an underlying theory that explains why our universe has the specific set of particles that it has. Supersymmetry is one of the more advanced accepted possible theories that try to explain why we have electrons, protons, and neutrons; why there are the quarks we see and not some other set. It is a complex theory I can’t pretend to say I understand, and now it is looking more and more like it is a theory that doesn’t work.
And that puts us back to having a universe that exists for reasons we just don’t know.
As confusing as dark matter is, regular matter isn’t always cooperative about being understood either. We have understood from a variety of observations that 5% of our universe should be made of the same normal (baryonic) matter that we, our computers, and our tables are made of. But we could only account for half of that amount in surveys that added up the mass of stars, planets, and other stuff in and around galaxies.
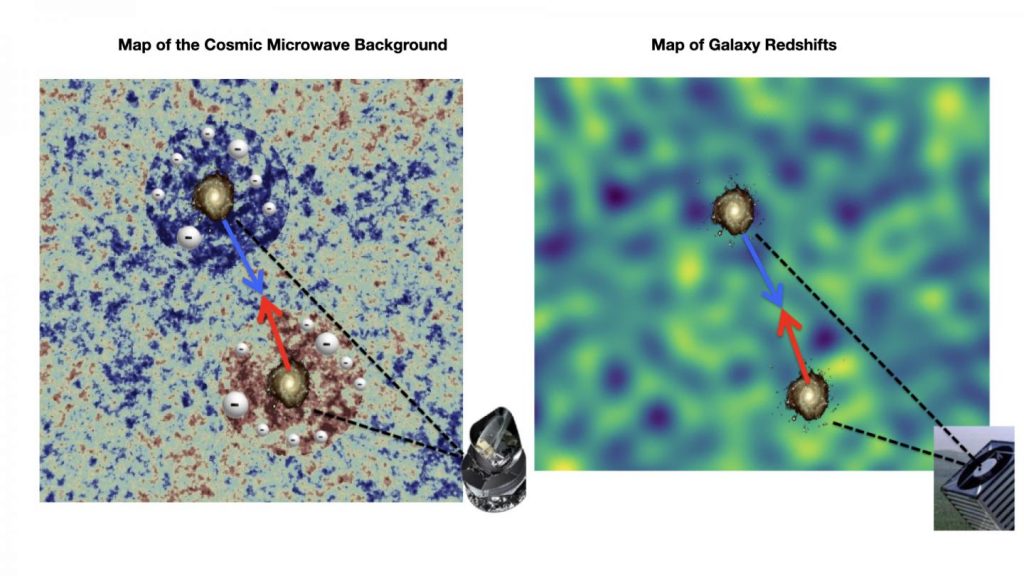
Now, a new study published in the Monthly Notices of the Royal Astronomical Society (MNRAS) in a trio of papers by four researchers — Carlos Hernández-Monteagudo, Jonás Chaves-Montero, Raúl Angulo, and Giovanni Aricò — looks purely at the velocities of galaxies to see how they gravitationally interact and uses this data to look for the pull of otherwise invisible gas. According to Jonás Chaves-Montero: Most of this ‘ordinary’ matter is invisible to us because it is not sufficiently hot to emit energy. However, by using maps of the redshifts of the galaxies we find that all of this matter fills the space between them.
To distinguish between dark matter and regular matter, they looked for the signature of electrons scattering light. These results look good, and I feel safe saying, half of the regular matter is hiding in the space between galaxies. And I’m ok with this. The universe is what is, and we’re going to learn about it one instrument, spacecraft, and computer model at a time.
For reasons I can’t explain, it sometimes seems like historic moments pile up around certain weeks of the year. We mourn in late January for too many astronauts lost. New Years’ and the Fourth of July celebrate the great landings and flybys of so many missions. And this weekend, inexplicably, brings us two anniversaries in planetary exploration.
On Monday, we celebrate the 1974 flyby of Mercury made by Mariner 10. If you try Googling this mission, you will learn the Mercury car line had its own Mariner edition, and I really hope this is a call back to this great little spacecraft that left the Earth before I was born.
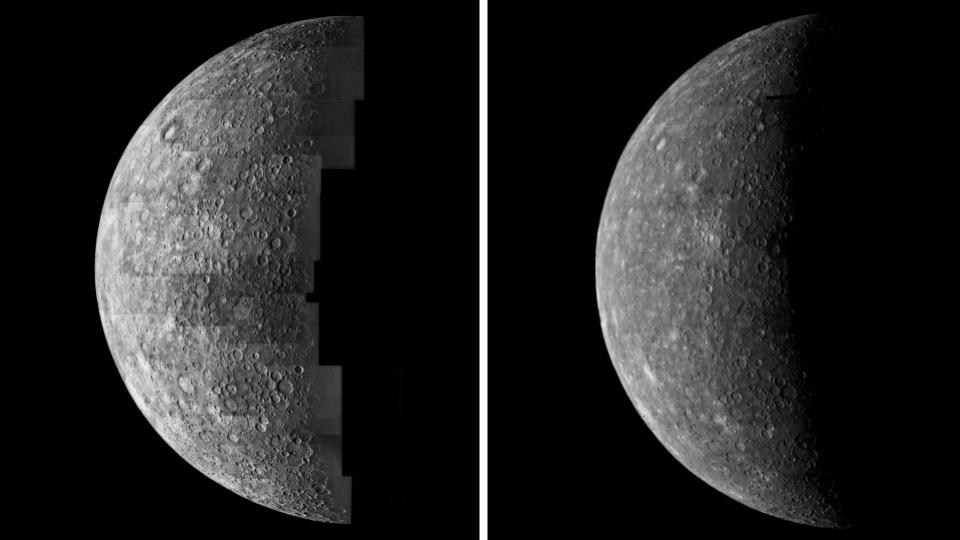
Mariner 10 was the last of its line of spacecraft. This series of missions made the first flybys of all the worlds in the inner solar system, entered orbit around another planet for the first time, and sent us back the crushing news that Venus is too hot for life and Mars is a desert world without canals or seas. Science fiction was forever changed by these missions, and the modern era of planetary science was started. From Mariner 10, NASA moved on to developing the Voyager and Viking programs, and the technology of these missions eventually evolved into the Cassini mission.
When Mariner 10 reached Mercury, cameras took what for 33 years would be our only closeup images of Mercury. Growing up, these images, mosaiced together with poorly matched backgrounds, filled my textbooks, and remarkably, they would go on to reveal new science thanks to modern image processing software like Photoshop. In the years leading up to MESSENGER’s arrival at Mercury, these images were reprocessed and revealed amazing details thanks to modern methods, showing that sometimes technology can reveal more than we imagined even in data we already have.
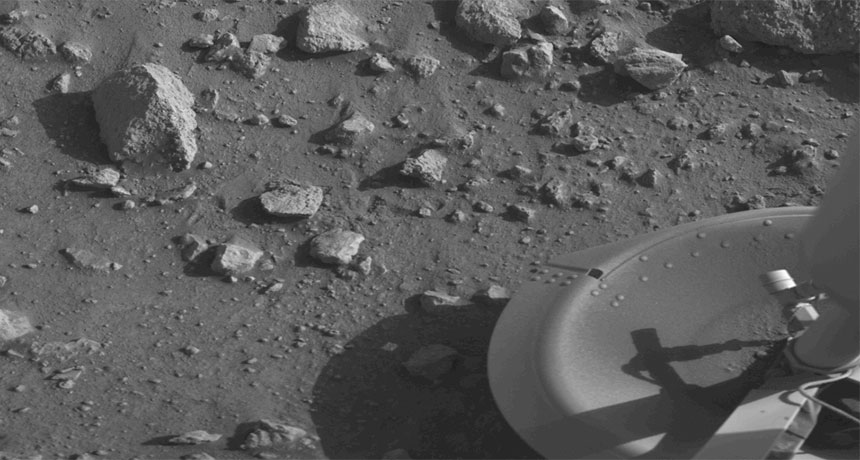
Tomorrow is the fiftieth anniversary of the start of Viking experiments to look for life on Mars. To be clear, those actual experiments occurred in 1976, but their origins go back to a 1971 lab experiment to see if conditions simulating those on Mars could produce organic compounds, and those experiments showed they could. Those results led to the inclusion of three different experiments on the Viking landers that looked to stimulate interactions with microbes in various ways; experiments that were inconclusive, with one researcher, Gilbert Levin, saying life had been found, and that in an analysis of images even lichens could be seen on surrounding rocks.
These results continue to be controversial. The result that seemed to indicate there was life is one in which they provided nutrients to the soil and looked to see if that soil outgassed materials that incorporated that stuff it had been fed. And if it did, here on Earth, that is a sign of life in the soil. And we saw this on Mars, but because the other experiments that were run did not also find life, it has been easier to simply say either life was not found or the results were inconclusive, leaving poor Levin to a lifetime of screaming to the wind, “Yes, there is life on Mars!” and a constant appeal to replicate these experiments – a replication that still hasn’t been done.
Today, the Perseverance rover is out there, not looking for these kinds of active signals and being able to interact with today’s life. It’s instead looking for fossils. But I hope someday soon, someday before Levin – who ran the experiment in 1976 – passes away, we will have further understanding of just what happened with his experiments that all started in a lab fifty years ago tomorrow.
This has been the Daily Space.
Learn More
Simulations Reconstruct Microscopic Clusters From Big Bang
- University of Göttingen press release
- “Formation of inflaton halos after inflation,” Benedikt Eggemeier, Jens C. Niemeyer, and Richard Easther, 2021 March 22, Physical Review D
Research Uncovers First Clues of Earth’s Supercontinent Cycle
- Curtin University press release
- “Archean geodynamics: Ephemeral supercontinents or long-lived supercratons,” Yebo Liu et al., 2021 March 22, Geology
Ocean Currents Predicted on Enceladus
- Caltech press release
- “A pole-to-equator ocean overturning circulation on Enceladus,” Ana H. Lobo et al., 2021 March 25, Nature Geoscience
Hidden Mechanism Revealed By Unusual Alaskan Earthquake
- Penn State press release
- “Triggering an unexpected earthquake in an uncoupled subduction zone,” Matthew W. Herman and Kevin P. Furlong, 2021 March 24, Science Advances
Not Looking Good for WIMPs as Answer to Dark Matter Mystery
- The dark matter mystery deepens with the demise of a reported detection (ScienceNews)
- Physicists’ devotion to symmetry has led them astray before (ScienceNews)
- “Annual Modulation Results from Three Years Exposure of ANAIS-112,” J. Amare et al., 2021 March 1, arxiv.org
“Missing” Baryonic Matter Found in Intergalactic Space
- IAC press release
- “Density weighted angular redshift fluctuations: a new cosmological observable,” Carlos Hernández–Monteagudo, Jonás Chaves-Montero, and Raúl E Angulo, 2021 March 25, Monthly Notices of the Royal Astronomical Society: Letters
- “Measuring the evolution of intergalactic gas from z = 0 to 5 using the kinematic Sunyaev–Zel’dovich effect,” Jonás Chaves-Montero et al., 2021 March 25, Monthly Notices of the Royal Astronomical Society
- “Tomographic constraints on gravity from angular redshift fluctuations in the late Universe,” Carlos Hernández–Monteagudo et al., 2021 March 25, Monthly Notices of the Royal Astronomical Society: Letters
A Look Back at Mariner 10, Viking 1, and the Potential for Life on Mars
- 50 years ago, experiments hinted at the possibility of life on Mars (ScienceNews)
- 40 years ago, Viking 1 pioneered U.S. exploration on Mars (ScienceNews)
- Mariner 10 info page (NASA)
- Viking 1 Lander info page (NASA)
- I’m Convinced We Found Evidence of Life on Mars in the 1970s (Scientific American)
Credits
Written by Pamela Gay and Beth Johnson
Hosted by Pamela Gay
Audio and Video Editing by Ally Pelphrey
Content Editing by Beth Johnson
Intro and Outro music by Kevin MacLeod, https://incompetech.com/music/