The IceCube Neutrino Observatory in Antarctica detected an electron antineutrino, confirming another piece of the Standard Model and proving that neutrino astronomy is feasible. Plus, a meteorite, wormholes, zodiacal light, and our weekly What’s Up segment.
Media
Transcript
Hello and welcome to the Daily Space. I am your host Beth Johnson and I am here to put science in your brain.
Today Pamela is off getting the first of her COVID vaccinations, and we wish her the best.
One of the tricks with this show is digging up news, and in digging up news today we found a bunch of science that actually required a fair amount of digging – or at least searching.
And it all starts with a flash. On February 28, a meteorite streaked across the sky over northern Europe and the United Kingdom. This particular streak was caught on camera from a bunch of different sites, and the combined imagery allowed researchers to figure out where the space rock came from and where any surviving pieces may have landed.
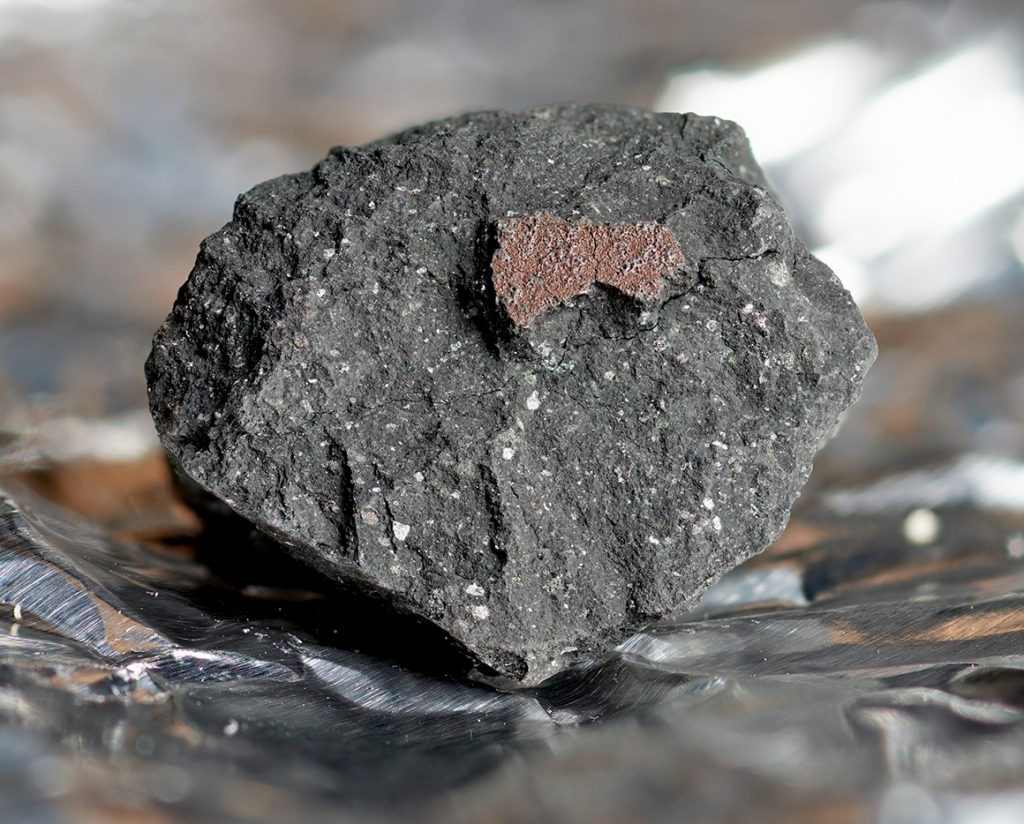
In this case, it landed in the driveway of a home in the small Cotswold town of Winchcombe. This was the first time in 30 years that a meteorite has been located in this way in the UK, and the particular bit that was found was a 300 gram carbonaceous chondrite fragment. This type of meteorite rarely survives to be identified on the surface of Earth.
According to Ashley King, who was among the first researchers on the scene: Nearly all meteorites come to us from asteroids, the leftover building blocks of the solar system that can tell us how planets like the Earth formed. The opportunity to be one of the first people to see and study a meteorite that was recovered almost immediately after falling is a dream come true!
Dr. Richard Greenwood captured the excitement of the moment, adding: I was in shock when I saw it and immediately knew it was a rare meteorite and a totally unique event. It’s emotional being the first one to confirm to the people standing in front of you that the thud they heard on their driveway overnight is in fact the real thing.
After being collected in Winchcombe, this fallen space rock was taken to the Natural History Museum in London where it will be further studied. This rock was recovered so quickly and in such pristine condition that this is essentially a free sample of an asteroid, not too different in quality from those brought back by Hayabusa2, although it will take a bit longer to sort exactly where in the solar system it came from.
Finding this asteroid fragment wasn’t that hard in the grand scheme of things, but the star, or rather the dead star, of our next story was more than a little surprising.
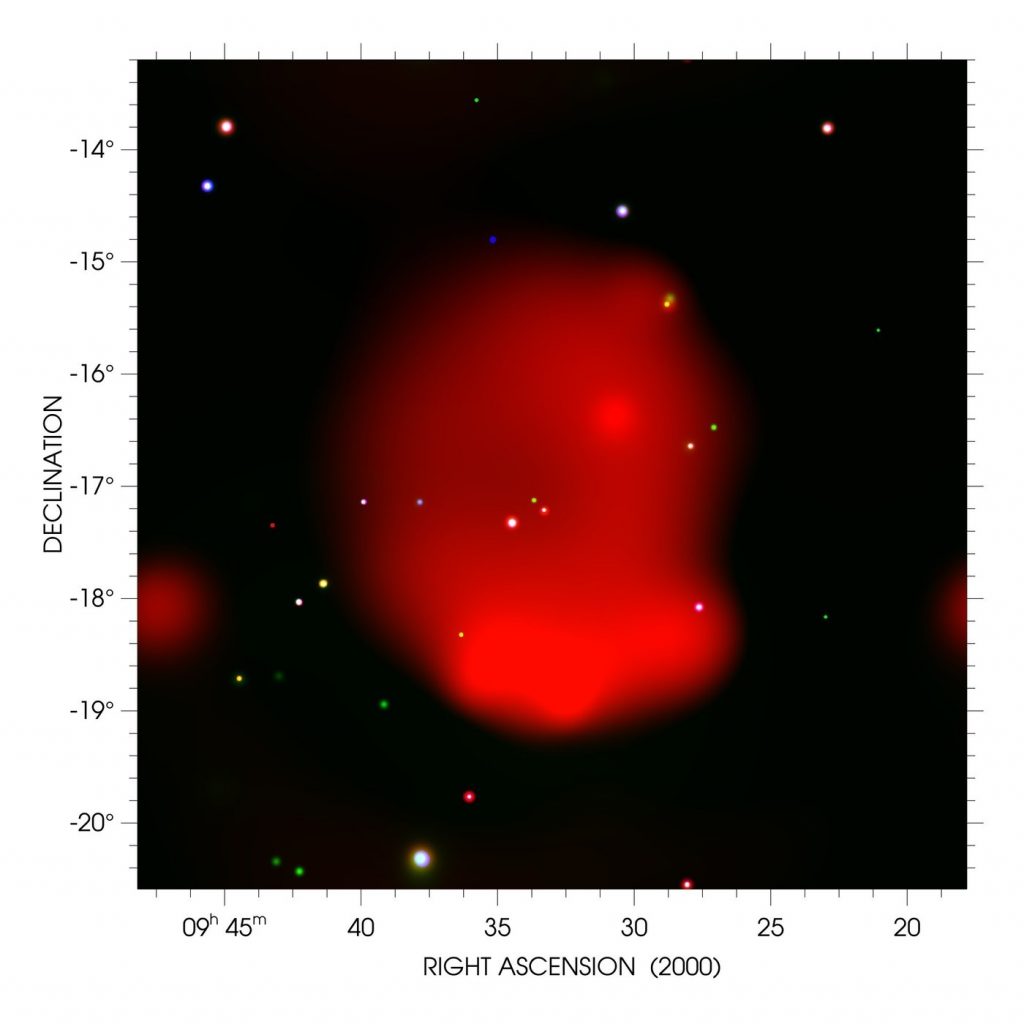
Just 500 parsecs away, a giant supernova remnant was hiding in plain sight. Invisible to your everyday telescope, this object shines in the X-ray sky with an apparent size of four degrees. This is a middle-aged remnant, that might appear like the Vela nebula if it were differently located. This object was found with the eROSITA All-Sky Survey and will be published in a new paper in Astronomy & Astrophysics. This research was led by Werner Becker, who named this object Hoinga, which happens to be the medieval name of Becker’s German hometown.
While it is a bit disturbing to realize the second-largest supernova remnant in the sky has been hanging out unseen for so long, it isn’t actually surprising. It’s estimated that galaxies like our own Milky Way should contain about 1,200 supernova remnants, but so far, we’ve only found about three hundred. The majority of these are invisible to optical telescopes and have instead been discovered with radio telescopes, and now with X-ray facilities. The eROSITA All-sky Survey is specifically designed to search supernova remnants like this one, and in the coming years, hopefully, we will find a lot more of the remnants hiding in plain sight, obscured by dust, gas, and light from other objects, or just faded away beyond our ability to see.
It’s really not possible to know what all exists out there, among the stars, beyond our ability to see. One of the most commonly chased wild ideas is the wormhole. Mathematically, wormholes were devised by Hermann Weyl in 1928, but the term wormhole was coined in a 1957 paper by John Wheeler and Charles Misner. For most of her career, Pamela has repeatedly explained that actual wormholes shouldn’t exist because they, according to the maths she learned, would collapse when any matter or energy entered them unless some otherwise unneeded form of exotic matter exists. The thing is until something is observed, there are always ways to tweak the maths and devise new possibilities.
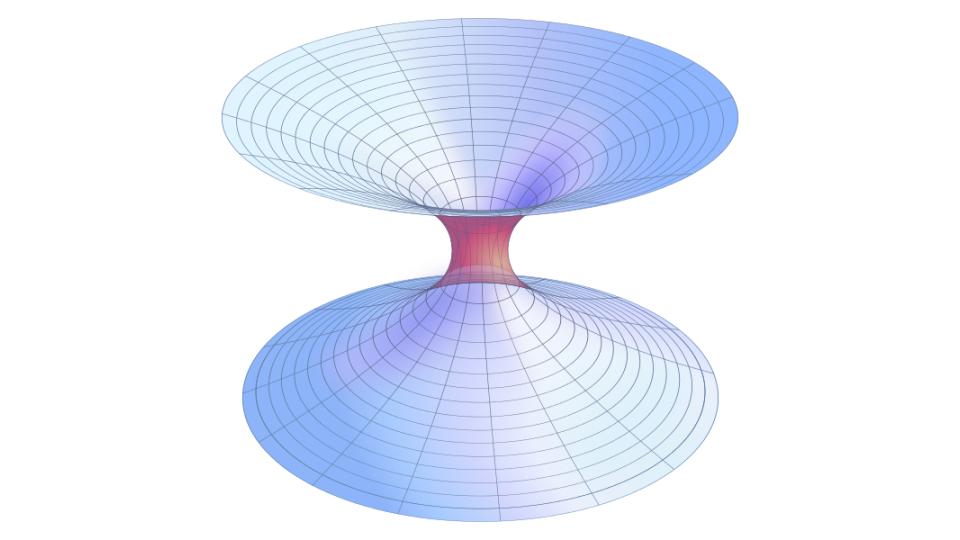
A new paper in Physical Review Letters by José Luis Blázquez-Salcedo, Christian Knoll, and Eugene Radu describes, and I quote, “a class of traversable wormholes… without needing any form of exotic matter.” This theory isn’t without a catch, however; their wormholes are constructed from just two massive particles. Specifically, two massive fermions, particles related to electrons or neutrinos but bigger.
And there is another catch: the matter passing through must have just the right amount of charge and mass. In this case, they devised a scenario where electrons are able to travel through a microscopic wormhole. Now, all because this is possible in maths, doesn’t mean it’s possible in reality, but there is nothing obvious to us or the peer-reviewers to say this kind of a wormhole can’t exist. I’m just not sure how we’re going to dig up these microscopic wormholes out there in the vastness of space, but that’s a problem for another day.
For today – we have one more study showing that the modern universe is expanding at 73-75 kilometers per second per megaparsec, a number that doesn’t agree with what is happening in the early universe. Ever since this discrepancy was discovered, folks have been trying to find either an error in the observations or some other factor that would bring the different sets of measurements into an agreement. The problem is, every different way we have found to measure things in the modern universe keeps matching. Supernovae results match lensing results, match – we learn now – the results of considering the average scatter in the brightness of stars in elliptical galaxies.
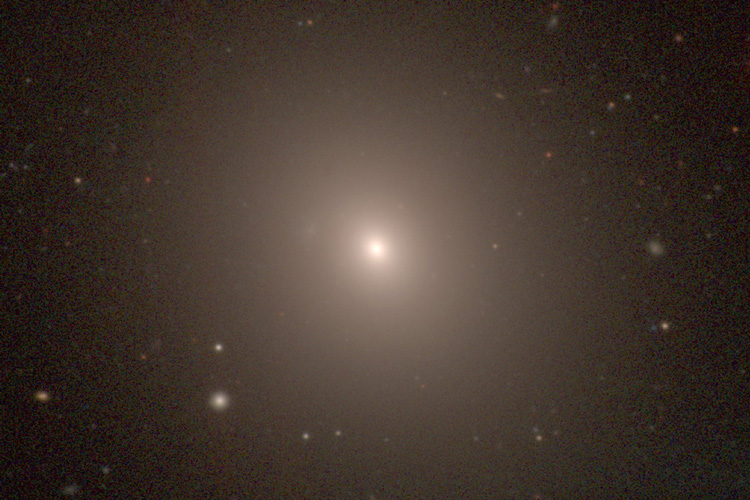
In a new paper in The Astrophysical Journal, a team led by John Blakeslee looks at 63 of the hundred most massive galaxies within 100 megaparsecs of our galaxy. These systems should have similar stellar populations, and closer systems will have greater pixel-to-pixel variations; essentially, we can clearly see their texture. Farther systems will appear smoother, as more stars blur together in each pixel.
Using these pixel-to-pixel variations, the team determined the distance to each galaxy. They also separately measured how fast each galaxy is being carried away from us by the expansion of the universe. Combining all this data and comparing it with other measurements, everything still matches, and we are no closer to understanding why the expansion rate of the universe is different when you look at different times in the universe.
It is all a kind of a hot mess; literally, the universe is hot, and it is messy.
Understanding our universe requires understanding how individual particles in the hearts of stars and in the first moments of existence have all been able to create the light that warms us and the particles that make up our planets, our technology, and ourselves.
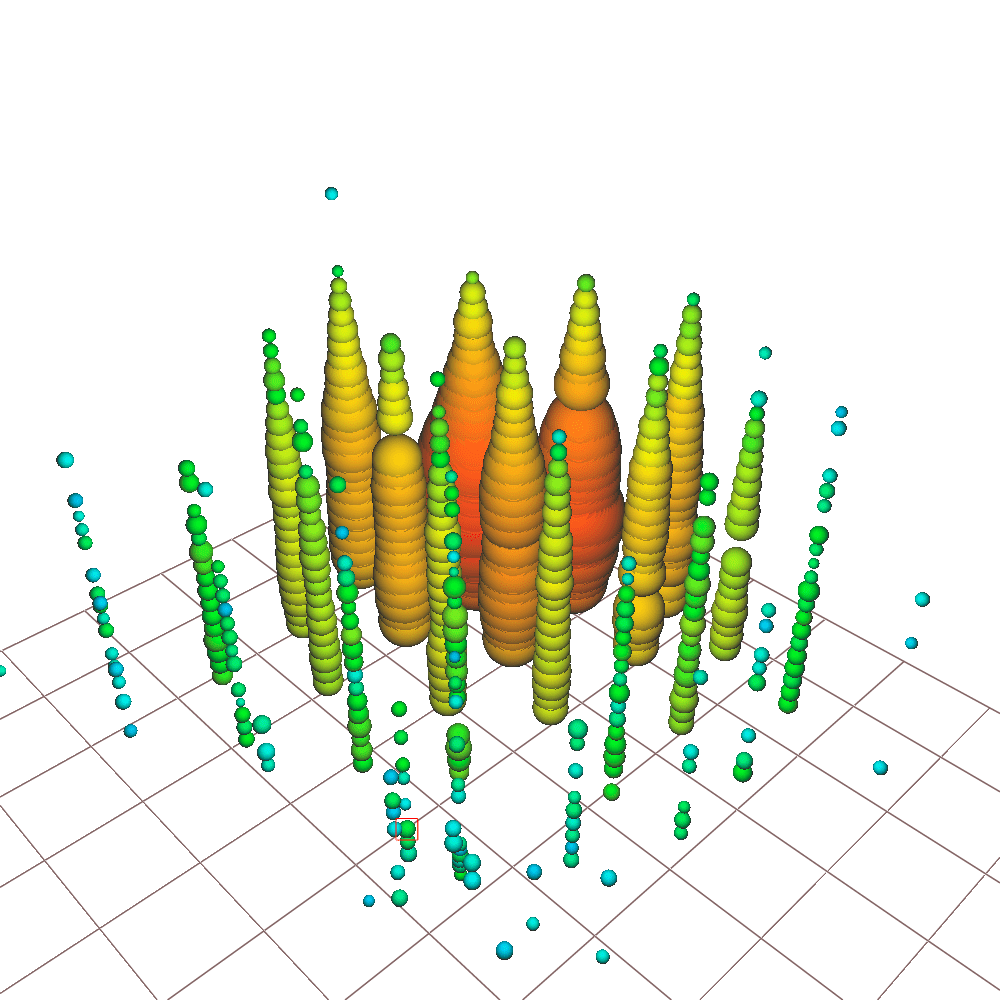
It’s hard to build massive telescopes, and the decade-long delay in the launch of the JWST is a case study of how hard it is to build scopes that allow us to see back to the earliest epochs of star and galaxy formation. On the other end of the scale, we build massive accelerators like FermiLab in Illinois and CERN in Europe, and we smash together particles after accelerating them to huge velocities, and out of the energy of the collisions, new particles can pop into existence. From these collisions, we’ve detected quarks, the Higgs boson, and myriad other particles. Unfortunately, there is a limit to what we can do, and some theorized reactions are beyond the capacity of our current engineering and our current power grid.
Back in the 1960s, Sheldon Glashow predicted that at the right energy, an antineutrino and electron could interact and produce a W-minus boson. This unstable particle plays a role in nuclear decay, and the W-, in particular, lets quarks change flavors, literally make down to become up, or at least the down quark to become the up quark. Every nuclear decay, for instance in cancer treatment or in the glowing radium hands of an antique watch, every nuclear decay is thanks to these particles. Now, we’ve been able to detect W- bosons with CERN and other accelerators since 1983. Electrons — Benjamin Franklin studied those. But anti-neutrinos are harder.
We do have various detectors that can broadly detect neutrinos and antineutrinos, but we can’t always figure out if we’re seeing a neutrino or antineutrino. Sheldon Glashow’s prediction of an antineutrino interacting with an electron and a W- boson offers a specific reaction that, if observed, will make it clear that an antineutrino is being seen.
It isn’t enough to mix these three particles together, however. The antineutrino in the reaction has to have just the right energy – an energy 1000 times greater than what CERN can produce. This means, to see this reaction, the universe must accelerate an antineutrino to just the right energy, fire that antineutrino in Earth’s direction and that particle must intersect with an electron inside a detector capable of seeing the interaction.
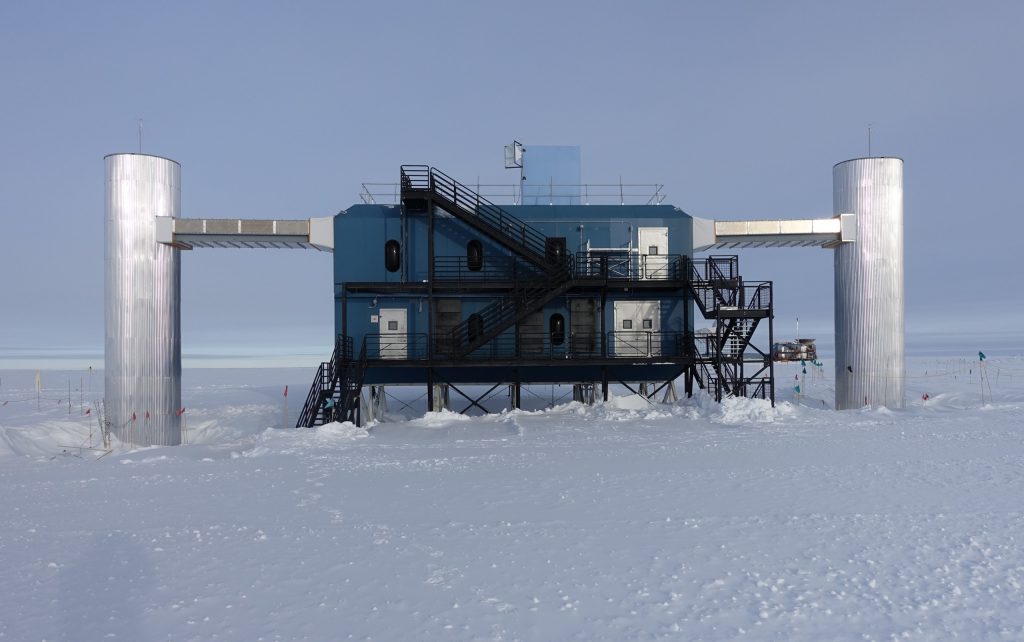
Basically, a lot of things in the universe have to precisely align. And on December 6, 2016, this is exactly what happened.
Down in Antarctica, at the Amundsen-Scott South Pole Station, there are thousands of sensors distributed over a cubic kilometer of ice watching for high-energy cosmic neutrinos and antineutrinos to interact and create cascades of particles and bits of energy. On that summer day, the specific cascade of particles distinctive of Sheldon Glashow’s resonance was spotted, signifying that a 6.3 petaelectronvolt antineutrino, likely accelerated by a massive black hole somewhere out in space, had precisely hit an electron.
This result is now published in the journal Nature by the IceCube collaboration.
According to researcher Lu Lu: Previous measurements have not been sensitive to the difference between neutrinos and antineutrinos, so this result is the first direct measurement of an antineutrino.
To me, this is amazing because so many things literally had to line up in time and space to allow this detection to happen, but because the universe is vast, the fact this has happened once means it should happen again and again and again. With each detection, we’ll be able to better understand the ratio of how many neutrinos and antineutrinos are out there being accelerated our way.
Just think: some of them could be passing through you right now, and you would never know. Science is weird. And it is wonderful.
I’d almost argue this story should be a part of our What’s Up segment this week since it’s about an astronomy phenomenon photographers look for. But it’s actually a science story about that phenomenon. I’m talking about zodiacal light. It’s a faint glow in the sky just before dawn or just after dusk, that happens when sunlight is reflected toward Earth through tiny dust particles that are orbiting the Sun. I learned that these dust particles are the result of comets and asteroids shedding material when their orbits get too close to the Sun.
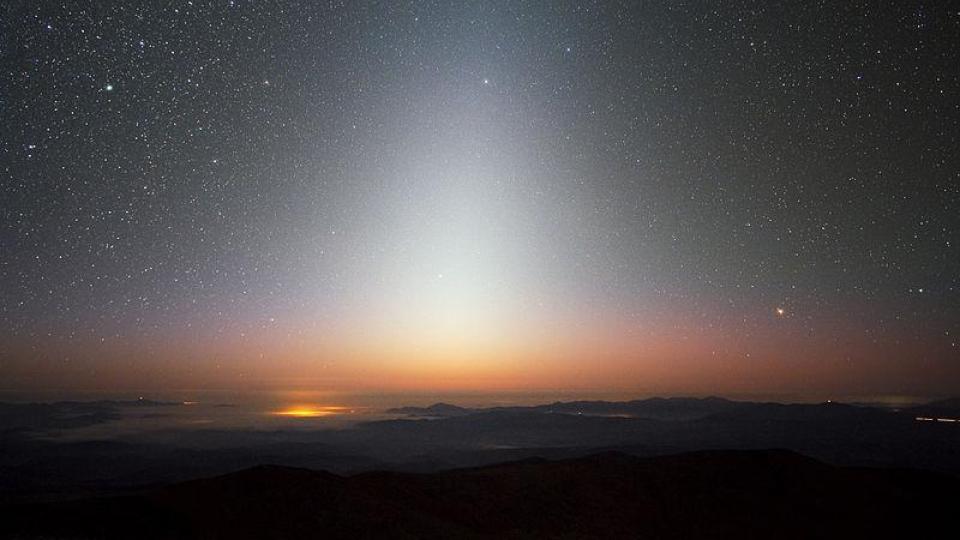
It’s basically the same cause as our meteor showers, except that we’re viewing the light through the dust from a distance instead of the Earth passing through the dust while we sky watch late at night.
Or so we thought. New research published in the Journal of Geophysical Research: Planets examines data from NASA’s Juno spacecraft that detected dust particle impacts as Juno traveled from Earth to Jupiter. The result is that the research team now thinks our zodiacal light is actually caused by dust particles from Mars.
The discovery was actually quite serendipitous, as the press release headlines claim. The instruments onboard Juno weren’t designed to find dust particles; they were designed to take images of the sky every quarter second. These images help determine Juno’s orientation in space by matching to star patterns, and that keeps the magnetometer’s measurements accurate. But lead author John Leif Jørgensen also programmed one of the cameras to report when an object was found in multiple images that didn’t match the catalog information. He was looking for undiscovered asteroids.
What he found was image upon image of tiny streaks appearing and then disappearing. After eliminating several possibilities, including a possible fuel leak from Juno, the team realized they were looking at interplanetary dust particles, and, due to Juno’s gravity assists, they were able to actually compile a distribution of those particles. The dust basically stretches from Earth to just beyond Mars. Earth, it seems, pulls in the closest dust particles, and that’s the cause of the zodiacal light. On the other end, Jupiter’s gravity grabs the particles.
The question remains, though, as to how Mars lost all these dust particles in the first place. It’s pretty interesting research that no one is really doing. In fact, research on zodiacal light is sparse enough that Dr. Brian May, of Queen fame, was able to complete in 2007 the thesis he started in the early 1970s because no one had really done anything in the field since. That thesis was titled A Survey of Radial Velocities in the Zodiacal Dust Cloud. Maybe he’ll help figure out this Mars question.
What’s Up
And now for another segment of “What’s Up”.
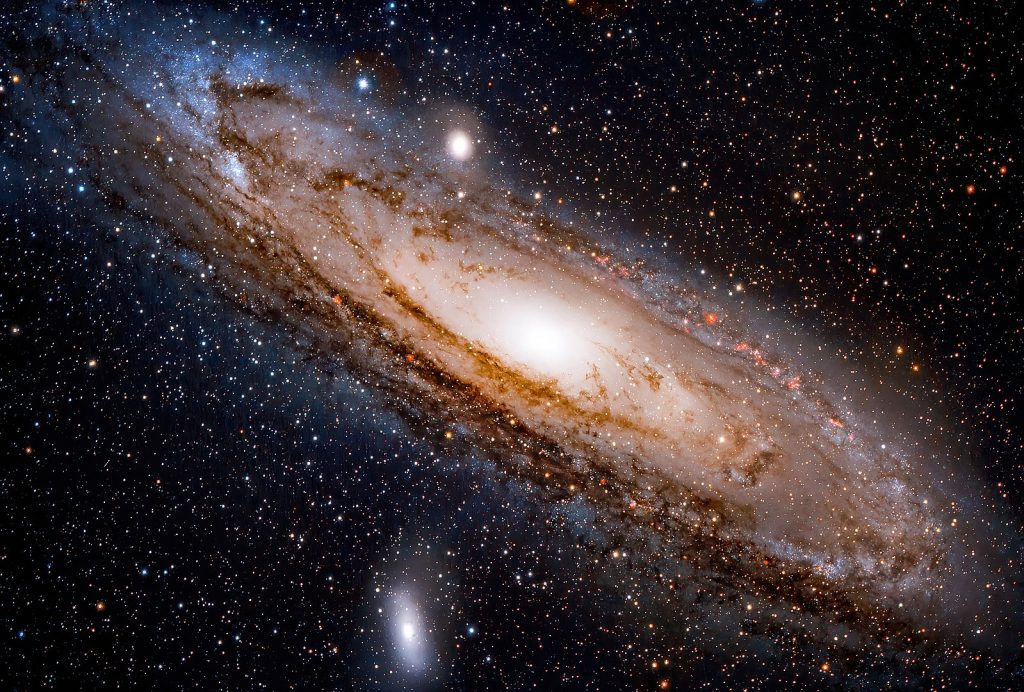
March is the beginning of what is called Galaxy Season. At this time of year, the Milky Way gets out of the way, making many galaxies easy to view in the northern hemisphere. Several impressive ones can be easily found in binoculars, including Messier 31, the Andromeda Galaxy. It is one of the easiest to find as it is quite bright. In binoculars, it looks like a great gray blob and if you have a telescope, you can tease out the dust lanes and other details the longer you observe it. M31 can be found easily: it is just up and to the right of the bright star Mirach in the constellation Andromeda. I usually use the Great Square of Pegasus to find it: it’s off to the left of the bottom left corner, above Mirach.
Another galaxy visible in binoculars is M81, Bode’s Galaxy. It is located in the northern sky about two-thirds of the way between the north star, Polaris, and a little bit to the left of the scoop of the Big Dipper. It can be resolved in binoculars but is best seen with a small telescope. Once you find M81, it should be easy to find M82, the Cigar Galaxy, since they’re really close together in the sky. For this pair, you’ll want to draw an imaginary line from the corner of the bowl of the Big Dipper below the handle (not next to) diagonally to the opposite corner, and then keep going about that same distance. It might take a few tries if you’re star hopping. I know it did for me.
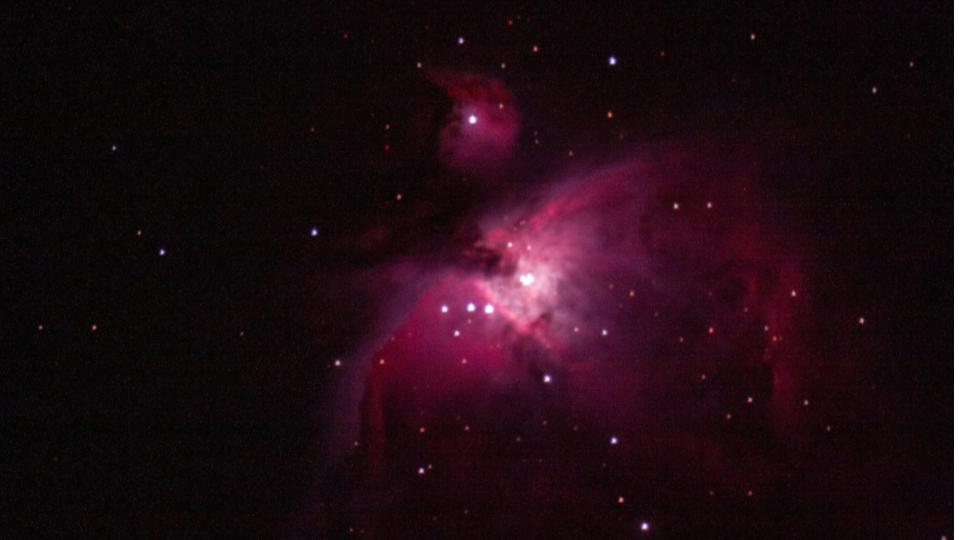
There is much to look at in the night sky besides galaxies. Take advantage of the new moon coming this weekend and once again check out the Great Orion Nebula, M42. Find the three stars of Orion’s belt, and then look for the sword hanging from the belt. In the middle of the sword is a bright cluster of objects, the largest of which is M42. It is very bright and puts on a good show in binoculars.
M45, the Pleiades star cluster, is also still visible. If the sky is very clear you will be able to see the six major stars of the cluster with your naked eye. You will see lots of stars in binoculars. Go from the belt of Orion to the ‘V’ of Taurus and keep going. You might even be able to pick out the faint nebulosity of the cluster.
Last, but not least, the planet Mars is going to be visible all month. Look for the bright pinkish-orange object in the western sky between sunset and midnight about a third of the way up from the horizon.
This has been the Daily Space.
Learn More
Meteorite Recovered After Fireball Over UK
- The University of Manchester press release
- Natural History Museum press release
Survey Finds Second Largest Supernova Remnant Using X-rays
- Max Planck Institute for Extraterrestrial Physics press release
- “Hoinga: A supernova remnant discovered in the SRG/eROSITA All-Sky Survey eRASS1,” W. Becker et al., to be published in Astronomy & Astrophysics (preprint on arxiv.org)
The Theoretical Possibility of Microscopic Wormholes
- University of Oldenburg press release (German)
- “Traversable Wormholes in Einstein-Dirac-Maxwell Theory,” Jose Luis Blázquez-Salcedo, Christian Knoll, and Eugene Radu, 2021 March 9, Physical Review Letters
New Expansion Measurements Calculated From Brightness of Elliptical Galaxies
- UC Berkeley press release
- “The Hubble Constant from Infrared Surface Brightness Fluctuation Distances,” John P. Blakeslee et al., to be published in The Astrophysical Journal (preprint on arxiv.org)
Antineutrino Detected Using IceCube, Proves Decades-Old Theory
- IceCube press release
- University of Wisconsin-Madison press release
- Michigan State University press release
- “Detection of a particle shower at the Glashow resonance with IceCube,” The IceCube Collaboration, 2021 March 10, Nature
NASA’s Juno Finds Earth’s Zodiacal Light Caused by Martian Dust Particles
- NASA JPL press release
- “Distribution of Interplanetary Dust Detected by the Juno Spacecraft and Its Contribution to the Zodiacal Light,” J. L. Jorgensen et al., 2020 November 11, JGR Planets
What’s Up: Galaxies, Orion Nebula, and the Pleiades
- Andromeda Galaxy star chart
- Bode’s Galaxy star chart
- Cigar Galaxy star chart
- Orion Nebula star chart
- Pleiades star chart
Credits
Written by Pamela Gay and Beth Johnson
Hosted by Beth Johnson
Audio and Video Editing by Ally Pelphrey
Content Editing by Beth Johnson
Intro and Outro music by Kevin MacLeod, https://incompetech.com/music/