Media
Summary
A massive black hole should be in the galaxy cluster Abell 2261. Observations with Chandra and Hubble haven’t located it. Plus, classifying supernovae with AI, neutron stars, the lithium composition problem, and more planetary science.
Transcript
This is the Daily Space for today, Friday, December 18, 2020. I am your host, Dr. Pamela Gay.
And I am your host, Beth Johnson.
And we are here to put science in your brain.
And sometimes, the science is just not as filling as one might wish. In our top story, we have news of a missing supermassive black hole.
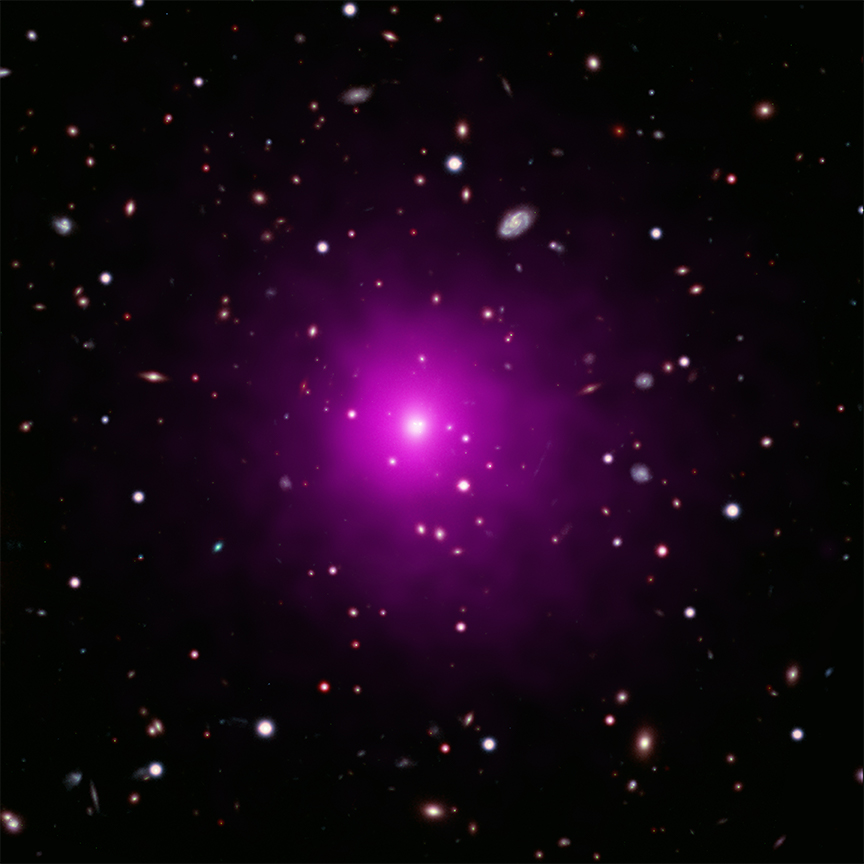
It is currently believed that all massive galaxies should have a black hole in their core that is proportional in size to the spheroid of that galaxy and the motions of its stars. While some small galaxies have been found without any obvious central black hole, massive galaxies have consistently had either obvious stellar motions or high energy emissions (or both) that are consistent with a massive object.
This was, at least, true until folks started looking at the central galaxy in the cluster Abell 2261.
Once upon a time, we referred to these systems as cD galaxies, and it was believed they were an outcome of how clusters form. Today they are more often referred to as giant elliptical galaxies and are thought more to be that first massive object that formed in the center of a dark matter halo in the early days of the universe.
In this revised picture, these galaxies should be just like any other elliptical galaxy, just bigger, and more likely to be actively merging with or consuming other systems. Astronomers, however, have been utterly unable to find high-energy, X-ray light coming from this system, and the motions of star clumps that can be resolved weren’t the definitive data that was needed either. In the radio, there was evidence of past emissions, roughly 50 million years ago but nothing indicating there is something there now.
While it’s entirely possible there is a supermassive black hole in the central galaxy of Abell 2261 that isn’t consuming anything, and thus isn’t giving off any X-ray emission; and while it’s possible this galaxy doesn’t have any close in, fast-moving star clumps we’ll be able to resolve well enough to use orbital motions to detect that supermassive black hole; while it is entirely possible there is a supermassive black hole in there that we just can’t detect… the fact that we can’t detect it has folks looking for an alternative explanation.
It’s thought that this galaxy has undergone a recent merger, and dynamically it is possible that the original supermassive black holes from each galaxy merged in such a way that they ended up rocketing off out of the system as gravitational waves were released in the opposite direction. There are aspects of this galaxy consistent with this story: the center of the galaxy has far more stars than is expected, and the densest part of the galaxy is 2000 ly off-center, which is extraordinary.
If — and the data here is not at all definitive — if it turns out that supermassive black hole mergers can asymmetrically release gravitational waves and send the newly formed supermassive black hole flying, then we should see more supermassive black hole-missing galaxies, and we also have a cool new thing to look for in our gravitational-wave data. Currently, detectors are only sensitive to smaller mergers, but the future has the potential to bring news systems sensitive to different kinds of events.
We don’t know exactly what is going on, and we can’t know with our current technology. The JWST may be able to reveal more details if it launches, but until then, we have a system with a secret. It may or may not have a central supermassive black hole, and either solution is scientifically awesome.
This research is described in a new paper accepted to the American Astronomical Society journals, and available on arXiv, with first author Kayhan Gultekin.
Researchers are teaching computers to science more and more every year, it seems. Machine learning is the “in” field these days, and in new work coming from the Center for Astrophysics at Harvard, scientists have taught an AI to identify and classify supernovae.
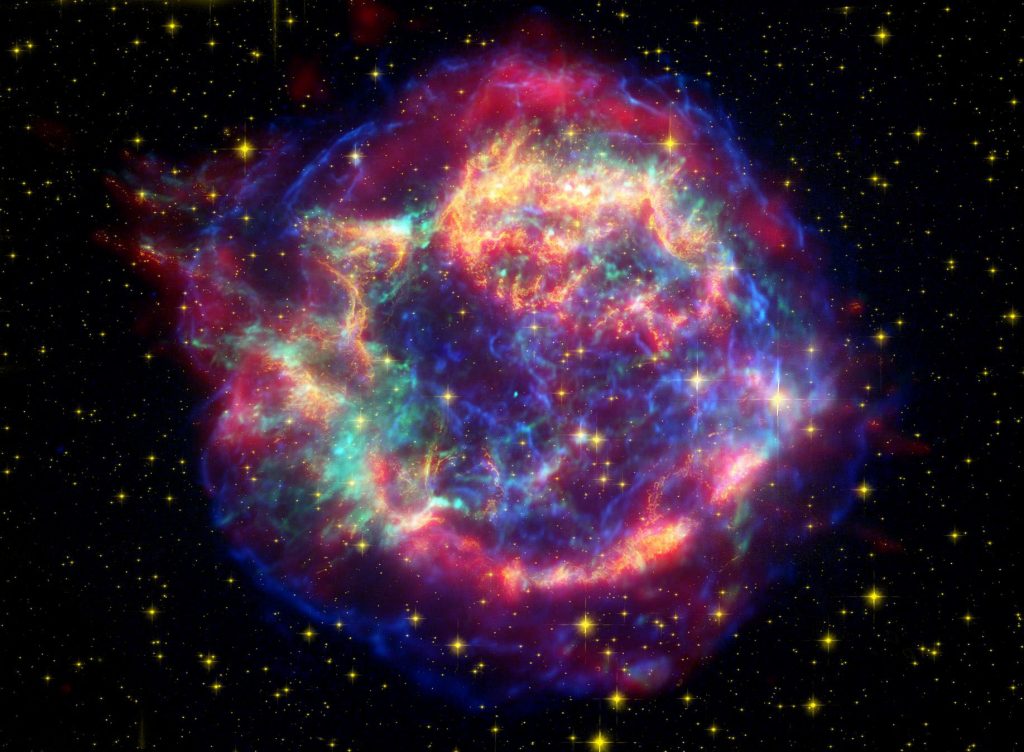
Using a data set of 500 novae with spectra from the Pan-STARRS1 Medium Deep Sky Survey, computers can now be used to find supernovae in observations that don’t have spectra. And it turns out that there are a lot of observations without spectra. So by using this existing data set of actual observations and not just simulated data, the researchers created an AI that can identify supernovae with stunning accuracy.
The Pan-STARRS1 Medium Deep Sky Survey had 2,500 known supernovae in its catalog, and since only 500 of those had spectra, that data set could be used to train the AI to answer specific questions. Edo Berger, an astronomer at the CfA explained, “The machine learning looks for a correlation with the original 500 spectroscopic labels. We ask it to compare the supernovae in different categories: color, rate of evolution, or brightness. By feeding it real existing knowledge, it leads to the highest accuracy, between 80- and 90-percent.”
This technique is going to prove even more useful once the Vera Rubin Observatory is online because while the Large Synoptic Survey Telescope [Ed. note: This name is defunct. LSST is the Legacy Survey of Space and Time] will surely discover a ton of new supernovae, the percentage we’ll have spectra for will not increase. Now scientists will be able to use all the other components of the observations to classify all those new novae we find. The corresponding papers for this work are published in The Astrophysical Journal.
High energy events, like supermassive black hole mergers, some supernovae, and the mergers of neutron stars all have the potential to give off massive amounts of energy via gravitational waves, while also sending out particles and light across many wavelengths. This triple feature of particles, light, and gravitational waves turns astronomy into a multi-messenger field, where we can start to look at things through a variety of instruments that probe radically different kinds of physics. This is all really new, and folks are still exploring all the ways they can leverage the combined information to understand our universe.
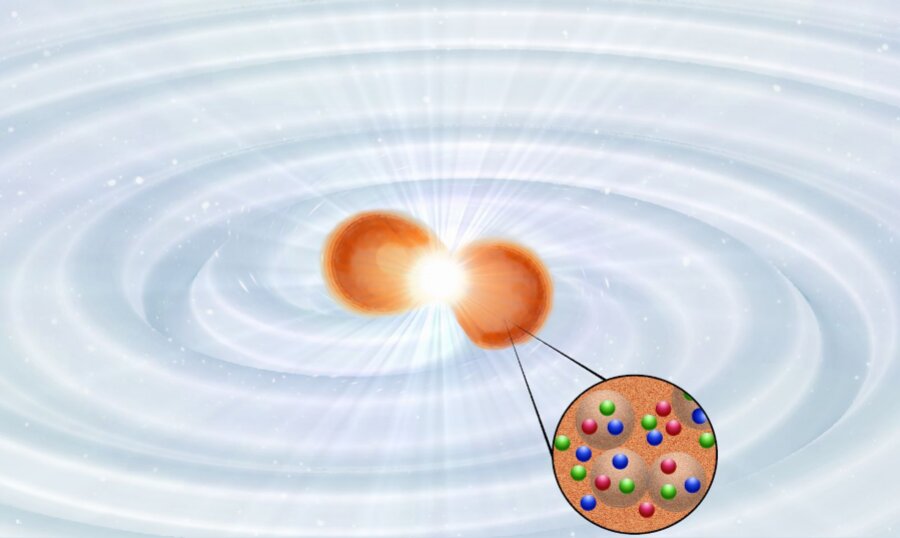
In a new paper in Science, led by Tim Dietrich, a team of astronomers examines how merging neutron stars may be used as standard candles, and what we can learn about the interiors of these weird stars from so many forms of data. So far, their estimates of neutron star sizes are in line with prior estimates made using pulsar data, and the error bars are such that it’s not yet possible to determine if neutron stars are solid neutrons or if their core is some denser, weirder substance that would allow them to have a smaller radius. It’s good to see consistency, and we can only hope to see the error bars coming down over time.
They also found, again with fairly hefty error bars, that the strength of detected gravitational waves can be combined with theoretical calculations about the initial energy in the waves, to sort out where the neutron star mergers were located. Essentially, if we know how much energy the wave should have contained at its origin, and we can measure how much energy it has as it passes through the Earth, we can calculate the distance. By combining thus calculated distances with measured redshifts of the galaxies containing the neutron stars, we can calculate how fast the universe is expanding. Preliminary results, with error bars, yield a value of 66 km/sec/Mpc and are consistent with measurements from all other techniques. While it’s frustrating that these results aren’t precise enough to say if the supernova-related expansion measurements or the Cosmic Mircowave Background-related measurements of the universe’s expansion are correct, again, things can only improve as more data is taken and techniques and instrumentation are perfected.
This is the start of a new area of astronomy. It’s exciting to see new techniques matching old results, and I can’t wait to see how multimessenger astronomy expands our understanding of the universe.
Did you know that there is a cosmological lithium problem? I did not, but a team led by scientists at the University of North Carolina at Chapel Hill did, and they may have taken the first steps to solve this problem in new work published in the journal Science.
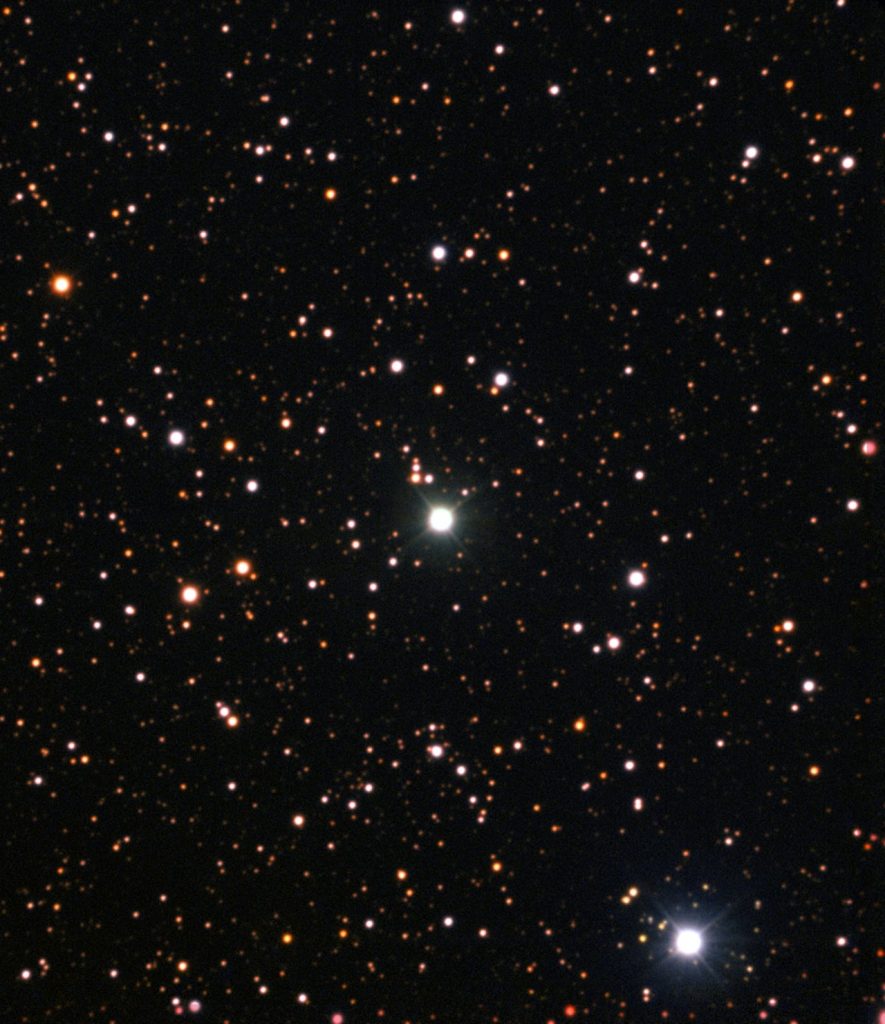
We use lithium for a host of important purposes, from powering cell phones to creating medicine. And we are pretty certain that much of the lithium in our universe was created during the Big Bang. But we haven’t been able to measure that lithium anywhere in the stars, and that is the problem.
Enter this team of researchers, who took spectra of two very old white dwarfs and found the crushed up remains of what were likely large asteroids. From the spectra, the team was able to determine the composition of those former rocky bodies, and they managed to measure both lithium and potassium for the first time.
J. Christopher Clemens, the designer of this new spectrograph, explained the importance of the discovery: Our measurement of lithium from a rocky body in another solar system lays the foundation for a more reliable method of tracking the amount of lithium in our galaxy over time
And lead author Ben Kaiser noted: Eventually with enough of these white dwarfs that had asteroids fall on them, we will be able to test the prediction of the amount of lithium formed in the Big Bang.
It’s always a good day in science when we have a breakthrough on a long-standing problem, so hats off to this team and their amazing work.
In what is becoming a recurring theme, we have a new story on how scientists thought something must act one of two ways, and the reality is both things can happen. In this particular case, we’re looking at volcanoes.
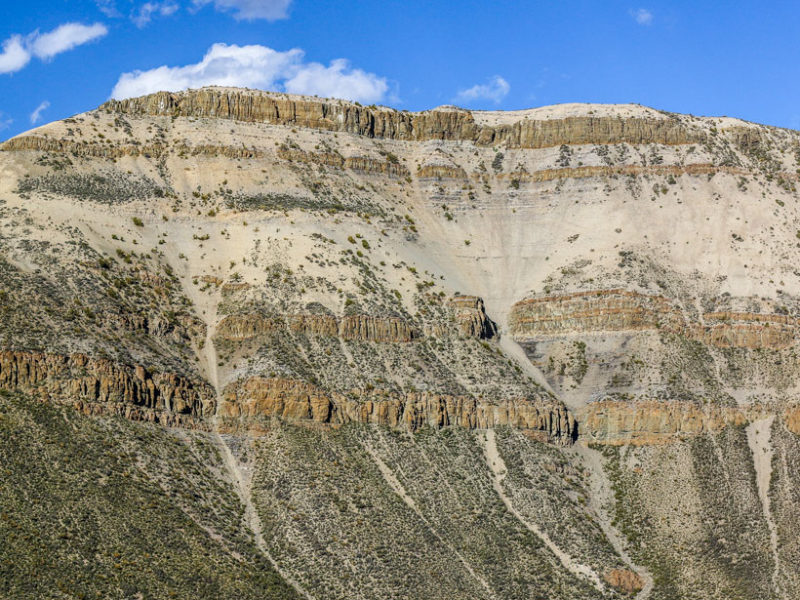
Trying to understand what exactly a volcano is likely to do has been of long interest but so far just hasn’t been all that possible. This is because volcanoes are complex systems that combine vast networks of reservoirs and tubes that store and transfer magma from deep in the Earth to the surface above. Understanding these networks is made all the more challenging because they are located underground, and even the most sensitive ground-penetrating radar doesn’t give us the kinds of resolution needed to completely understand exactly how magma pushes its way through the rock.
Luckily, erosion and plate tectonics can help; there are various places on our planet where the guts of volcanoes have been exposed on cliff faces and in volcanic fields.
In order to build a new and better understanding of volcanoes, an 85-person workshop was convened in Argentina that combined two days of scientific meetings and a three-day field workshop that looked at rock formations altered through magma intrusions and other volcanic features.
Of interest was how rocks deform when magma encounters them – specifically are they cut like with a knife or are they pushed like with a probe. In the knife scenario, magma cuts into a rock that then tears and splits out of the way. In the probe scenario, the encountered rock compresses and deforms as it is pushed along. It turns out that both these situations happen — sometimes magma cuts through rock and sometimes it pushes — and this has consequences for how we model volcanoes and what we look for. Put simply, there is no one way that volcanoes store magma, and when we see the ground begin to bulge, all we can know is something is moving and not if something is gearing up to explode.
These are not satisfying answers. This does not help say when or for how long a volcano may erupt. But this does tell us we need to model things in more ways than one, and that our predictions need to say “If this, then that, and if this other thing, then, well, something different.”
Our planet is complex. And the data looked at in this research is complex. The report from this research conference has now been published in Eos and includes numerous references to individual papers that discuss different aspects of this work in detail.
The data analyzed combined our standard, gather-what-your-grant-allows, academic data with much more thorough data obtained with more expensive equipment and larger data gathering budgets by petroleum companies. With this additional information, researchers were able to apply their theoretical knowledge to gain new insights that are leading to new laboratory experiments to try and model what’s going on at a much smaller scale. I can only hope that we see more of these joint industry and academia ventures in the future.
I seem to be talking a lot about dust this week. It’s an important issue in a lot of our planetary science research; however, we mostly look at dust and its physical effects, like erosion or air quality or glacial stability. And now it turns out that we may be overlooking a very important effect — an electrical effect.
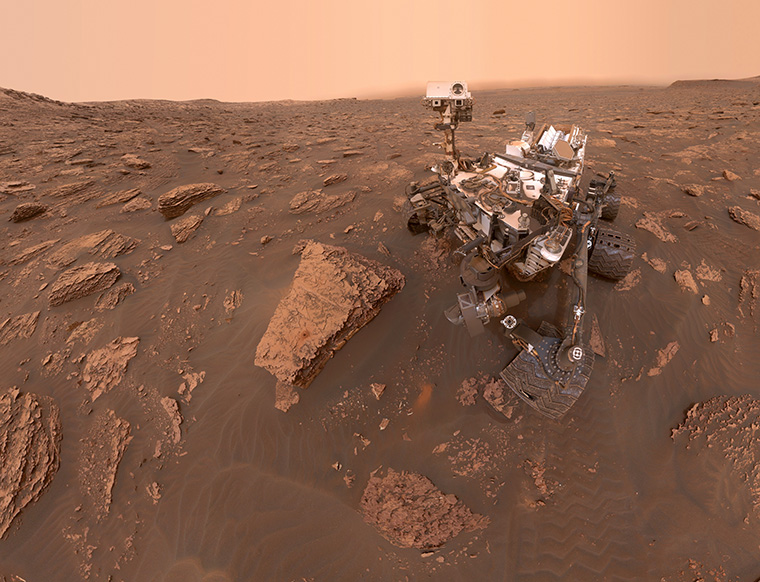
According to new research from Washington University in St. Louis (WUSL) and published in the Journal of Geophysical Research: Planets, electrical effects can affect the chemical composition of a planetary body’s surface and atmosphere in a relatively short time. The study focuses on amorphous sulfur and chlorine salts found by the Curiosity rover at Gale Crater on Mars, and, per the press release: The chemical signature of these materials could have been induced by electrochemical processes during Martian dust activities in a relatively short geologic time frame: years to hundreds of years.
These electrochemical processes could cause changes in the crystal structure of minerals, removing water and causing the oxidation of sulfur, chlorine, and iron. This research should be used to make further decisions about our research priorities in upcoming Mars rover missions, and it’s suggested that we look to the subsurface of the red planet, where we could find ancient materials that might even have evidence of biomarkers. I’m all for that.
And finally, because it’s that time of year, the European Space Agency (ESA) has provided us with a seasonal image of Mars — an angel and a heart. These formations were spotted by ESA’s Mars Express near Mars’ south pole back in November.
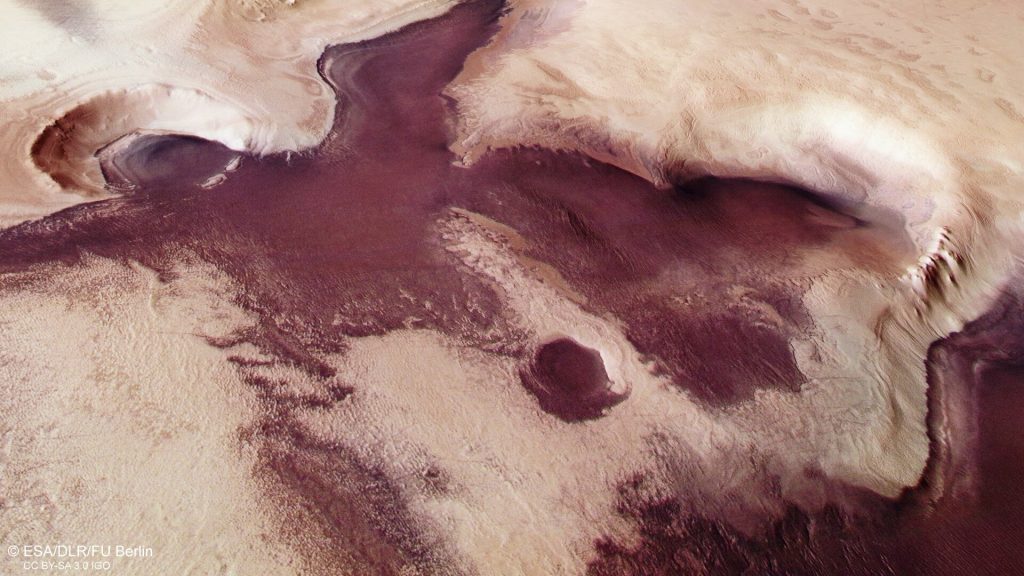
On a less whimsical note, the red in these images is caused by the composition of the sand dunes — namely sands that have a lot of dark, rock-forming minerals such as pyroxene and olivine. And since it’s summer on Mars, the amount of polar ice is at its lowest, revealing all that gorgeous dark red sand.
There’s even more cool science involved here! The angel’s hand may be a sublimation pit where the ice has undergone rapid sublimation and left a divot on the surface. The halo and head are an impact crater. And while the formation of the heart is something of a mystery, scientists think it may have existed deeper below the surface in layers of material formed by ancient volcanic activity and been revealed by erosion over time.
Also really interesting is the dark patch of crisscrossed lines off to the left side of the image. Those are made by dust devils. So we have something for everyone in this image: love, hope, and a little devilish work. Happy holidays, everyone!
This has been the Daily Space.
Learn More
Abell 2261: On the Hunt for a Missing Giant Black Hole
- Chandra X-ray Observatory press release
- “Chandra Observations of Abell 2261 Brightest Cluster Galaxy, a Candidate Host to a Recoiling Black Hole,” Kayhan Gultekin et al., to be published in AAS Journals (preprint on arxiv.org)
Artificial Intelligence Classifies Supernova Explosions with Unprecedented Accuracy
- Center for Astrophysics press release
- “SuperRAENN: A semi-supervised supernova photometric classification pipeline trained on Pan-STARRS1 Medium Deep Survey supernovae,” A. Villar et al, 2020 December 17, The Astrophysical Journal (preprint on arxiv.org)
- “Photometric Classification of 2315 Pan-STARRS1 Supernovae with Superphot“, G. Hosseinzadeh et al, 2020 December 17, The Astrophysical Journal (preprint on arxiv.org)
Gravitational Waves Probe Exotic Matter inside Neutron Stars
- Los Alamos National Laboratory press release
- Scientific American article
- “Multimessenger constraints on the neutron-star equation of state and the Hubble constant,” Tim Dietrich et al., 2020 December 18, Science
Can white dwarfs help solve the cosmological lithium problem?
- Eureka Alert article
- “Lithium pollution of a white dwarf records the accretion of an extrasolar planetesimal,” B. C. Kaiser et al., 2020 December 17, Science
A Fresh Perspective on Intricate Volcanic Plumbing Systems
Powerful electrical events quickly alter surface chemistry on Mars and other planetary bodies
- WUSL press release
- “Amorphization of S, Cl‐Salts Induced by Martian Dust Activities,” Alian Wang et al., 2020 November 17, Journal of Geophysical Research: Planets
Festive features spotted near Mars’ south pole
Credits
Written by Pamela Gay and Beth Johnson
Hosted by Pamela Gay and Beth Johnson
Audio and Video Editing by Ally Pelphrey
Content Editing by Beth Johnson
Intro and Outro music by Kevin MacLeod, https://incompetech.com/music/