Media
Transcript
When labeling astronomers, we generally fall into a very small number of categories. There are the observational folks, the computational folks, the theorists, and the instrument builders. That’s most of us, and many of us will wear more than one of those labels during our lifetimes. Our combined efforts build the instruments that are used to observe our skies and test our theories against reality, and when those datasets get big enough and the theories get complex enough, we throw computational might into the problem.
But sometimes our computers just can’t handle the complexities of the universe in the needed detail. The interactions of myriad particles in planetary atmospheres and the propagation of shock waves in supernovae are all problems that we can approximate in computers, but our approximations just don’t look like the reality we observe. Situations like these are when we turn to one of the rarest kinds of astronomer – the laboratory astrophysicist. These folks build equipment that simulates the specific conditions of different astronomical events and recreates our universe in a box, or in the case of today’s top story, in a pizza-shaped wedge.
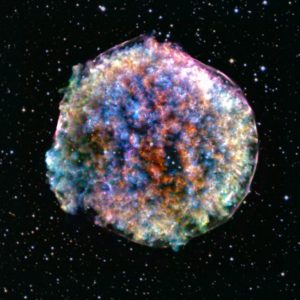
The Hubble Space Telescope and other high-resolution systems have allowed us to see supernovae remnants as complex lumpy, bumpy systems that are each unique. Trying to recreate the complex leading edge of these systems has proven more than a computer model can handle. Now though, there is a laboratory option. Researchers at Georgia Tech have created a supernova simulator in their lab. Like computational simulators, it only looks at a section of the explosion – a wedge expanding away from a simulated core – but this system provides enough three-dimensional particle interactions to recreate supernova physics in a way that has previously just not been possible.
In an actual supernova, the core of a star will collapse after it runs out of fuel to power the light-producing nuclear reactions that normally support the star. This collapse triggers a powerful explosion that pushes the high-density star material in the inner regions of the star outwards, where it mixes violently with the surrounding lower density materials. These layers have fairly defined boundaries, and in this supernova simulator, this system is recreated as an actual explosion that pushes up through the two different layers of high-density and low-density gas. According to the press release: Heavier gas in inner layers stabs turbulent outcrops into lighter gas in the outer layers. Then behind the blast wave, pressure drops, stretching the gases back out for a different kind of turbulent mixing. The final structures of the supernova are shaped in part by the naturally forming irregularities at the boundaries between the two densities of gas. These perturbations are stretched and skewed at angles when blasted by the explosions shock wave.
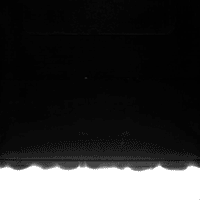
The Supernova-in-a-Wedge machine allowed researchers to take high-speed images of what was going on at the density boundary and capture the formation of these iconic supernovae structures. Along with the regular bumps and bubbles, they also observed turbulent spikes of high-density gas pushing into the low-density region, and bubbles of light gas trapped inside areas of high-density gas.
In addition to defining how these shockwave-driven structures form, this physical simulator allows researchers to better understand the timescales of these structures’ evolution. This will ultimately help us better date the formation of observed supernova remnants.
This is cool research that makes it clear that sometimes you need to try and recreate what you see, and it is only in that recreation that you can understand what you see.
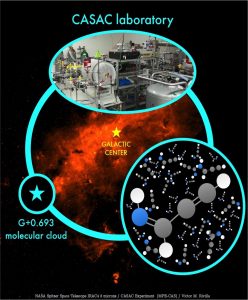
Laboratory science doesn’t always go in that direction, however. Sometimes we see things in the lab that we need to go searching for in the universe. In our ongoing search for the origins of the stuff of life, scientists have worked to understand what molecules life needs and to then see where they can be formed. One of the more fundamental molecules in life as we know it is amino acids. These molecules are primarily composed of carbon, nitrogen, oxygen, and hydrogen, and the human body uses twenty different amino acids in its various functions.
Amino acids have been observed in interstellar molecular clouds since 2003, and in a new paper accepted in Astronomy & Astrophysics, researchers have gone looking in the lab for the precursor molecules to amino acids and having found those molecules, they’ve turned around and looked in astronomical data where they have found those precursor molecules in space. The molecule in question is propargylimine, and it has been found in the molecular cloud G+0.693-027, which is located in the direction of the galactic center. The chemistry behind these molecules can take place in cold and icy areas, including on the surface of icy dust grains and even on the surface of asteroids or within comets. While we benefit from warmer production methods of these molecules, it’s intriguing to see that the building blocks of life could have been brought here rather than made here.
For our final story of the day, I’d like to call your attention to some particle science results that we’re going to be following.
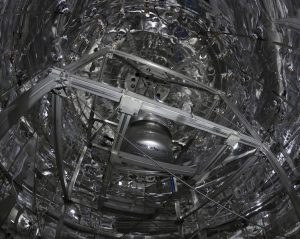
Scattered around our world are a variety of underground detectors that are protected from the random noise of space and are tuned in to look for the specific flashes of light that can occur when particles like neutrinos collide with specific liquids. One particular detector, the XENON1T in Italy is filled with ultra-pure liquid xenon. Designed to search for certain theoretical kinds of dark matter, it has so far found none of the particles it hoped for, but it has found a weird background of particle interactions that weren’t expected.
While there is a chance that what they are seeing is the noise created by a few stray tritium atoms in their detector, they could also be observing some kind of a previously unobserved particle, like an axion from the Sun, or they could be seeing an effect caused by neutrinos having a higher magnetic moment then currently expected. Since it would only require a few tritium atoms for every 10^25 xenon atoms to cause this effect, I’m not going to suggest it’s time to get excited about potential new physics. It’s still cool though to see discussed the possibility that axions are being detected as noise in a dark matter detector while dark matter isn’t detected. We’re pretty sure this result will turn out to be absolutely not exciting, but just in case it is, we’ll be keeping an eye on how this story evolves.
For now, though, this is all the science we have for today.
Learn More
This Supernova in a Lab Mimics the Cosmic Blast’s Splendid Aftermath
- Georgia Tech Research Horizons article
- “Supernova Hydrodynamics: A Lab-scale Study of the Blast-driven Instability using High-speed Diagnostics,” Benjamin Musci et al., 2020 June 17, The Astrophysical Journal
New Organic Molecule Discovered in Interstellar Cloud
- Max Planck Institute for Extraterrestrial Physics (MPE) press release
- “Propargylimine in the Laboratory and in Space: Millimetre-wave Spectroscopy and First Detection in the ISM,” L. Bizzocchi et al., 2020, to appear in Astronomy & Astrophysics (Preprint on arxiv.org)
Observation of Excess Events in the XENON1T Dark Matter Experiment
- Kavli Institute for the Physics and Mathematics of the Universe (Kavli IPMU) press release
- “Observation of Excess Events in the XENON1T Dark Matter Experiment,” XENON Collaboration, 2020 June 17 (Preprint on arxiv.org)
Credits
Written by Pamela Gay
Hosted by Pamela Gay
Audio and Video Editing by Ally Pelphrey
Content Editing by Beth Johnson
Intro and Outro music by Kevin MacLeod, https://incompetech.com/music/