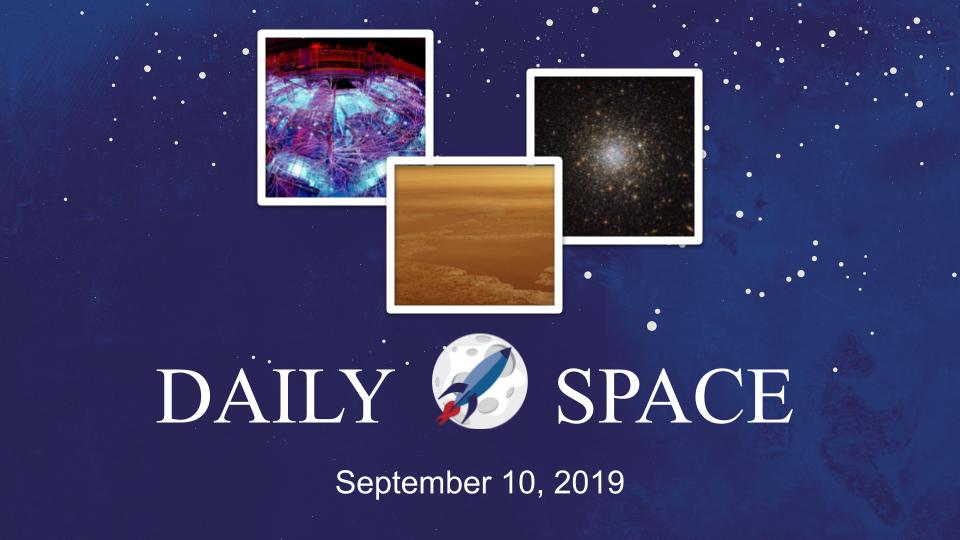
Join us as we explore the insides of stars, dynamics of star clusters, and lake formation on Earth and Saturn’s moon Titan. Over and over we see the same physics works the same way everywhere, and still finds ways to surprise us!
Links
The Effects of Iron on Stellar Nuclear Reactions
- Sandia experiments at temperature of sun offer solutions to solar model problems (press release)
- Systematic Study of L-Shell Opacity at Stellar Interior Temperatures (Physical Review Letters)
Dynamical Ages of Star Clusters
- Hubble Explores the Formation and Evolution of Star Clusters in the Large Magellanic Cloud (press release)
Explosive Lake Formation on Titan
- New Models Suggest Titan Lakes Are Explosion Craters (press release)
- Whoa. Lakes on Titan Might be the Craters from Massive Underground Explosions (Universe Today)
Transcript
Today’s news takes us far and wide but starts in a laboratory at Sandia National Labs, just outside Albuquerque, New Mexico. This experiment, the Z-machine, is a pulsed energy experiment that allows researchers to recreate the 4.1million degree Fahrenheit conditions deep in the heart of the sun.
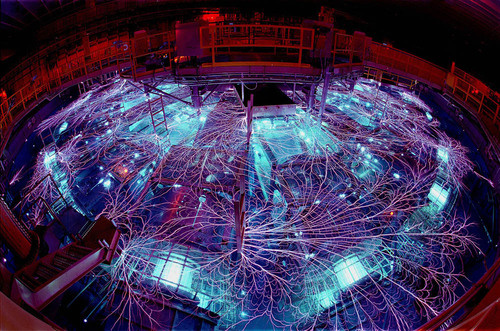
(Image Credit: Randy Montoya)
Our models for how and when nuclear reactions take place, and how that energy radiates have previously relied on 4 measurable qualities of a star: mass, radius, surface temperature, and atmospheric composition. While these factors put a lot of constraints on our theories, this just isn’t enough data to allow us to test all our assumptions. Some of the hardest to understand issues involve how complex atoms like iron interfere with nuclear reactions by absorbing and re-emitting light. In astronomy, we refer to all atoms more complex than Hydrogen and Helium as metals and the amount of these atoms in a star as the stars metallicity.
These heavier atoms act, to use a stretched analogy, like cooling rods in a nuclear power plant. They block the transmission of energy from one place to another, and thus limit the rate of reactions. Now, atoms like Iron don’t block all the energy – They aren’t completely opaque. if they were our Sun couldn’t have nuclear reactions. The question has been, just how much energy do they block. For decades, we’ve made assumptions based on what we know from lower temperature experiments and quantum mechanics, … and also from what we’ve learned from Nuclear Bombs and other more controlled nuclear processes. Unfortunately, those estimates and our stellar models haven’t meshed together to recreate the Sun we observe. This means either our stellar model has been wrong, or our understanding of atomic opacities has been wrong.
Using the Z-Machine, scientists at Sandia have for the past ten years systematically vaporized Iron samples and measured how actual, super-heated iron plasma blocks energy at the temperatures in our Sun. In a new paper in the journal Physics Review Letters, a team led by Taisuke Nagayama finds that Iron limits reactions much more than had previously been understood. By adjusting computational models for the Sun to take into consideration these new measured opacities, they find the model gets one step closer to matching the actual sun.
While our overall models for how stars work seem to be turning out ok, this difference between prediction and reality concerning iron’s opacity implies that our understanding of atoms is somewhat flawed. To try and model the atoms’ actions, physicists are turning to new models that explore the possibility that iron can absorb more than one photon at a time. While that seems like no big deal, sure, an atom can absorb 2 photons, this is actually a really weird solution because our 1 photon models for nickel and chromium actually match reality as measured with the Z-machine.
This is a complex problem – one that looks at the inner workings of atoms and expands out to dictate the behaviors of stars. This work, the result of 10 years effort, is just a start. To quote project lead Nagayama “The science impact is enormous — this obligates us to continue examining the experiment’s validity.”
When I was in graduate school, I often heard people working on problems in cosmology declare that stellar astronomy wasn’t worth pursuing because stars are a solved problem. Today’s news makes it clear that even 2 decades later, stars and the structures they form are far from understood.
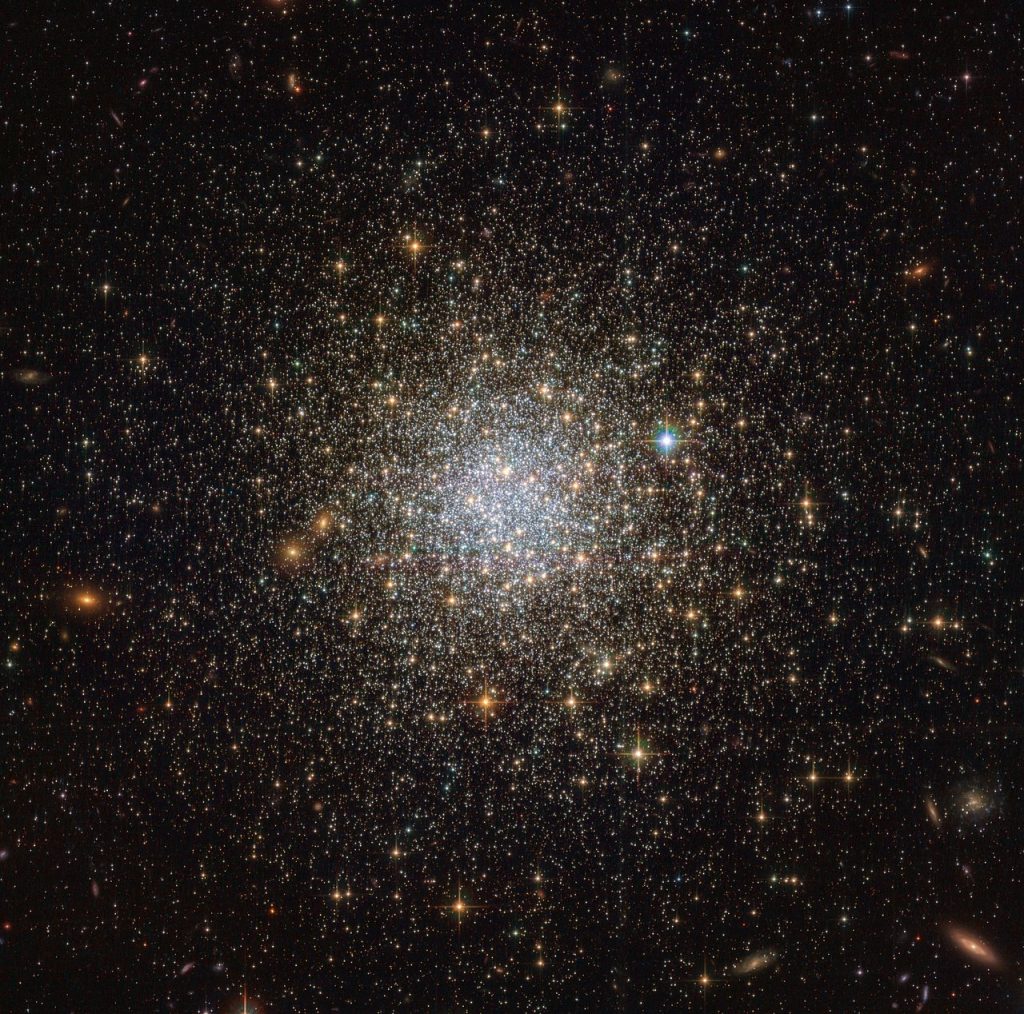
Credit: ESA/Hubble & NASA
One of the best ways we have to understand stars is to understand star clusters. From looking at the stellar nurseries we call open clusters, to looking at the aged stellar retirement homes we call globular clusters, we can use clusters to look at how a collection of stars that all formed at about the same time and out of the same mix of materials will evolve over time. Our focus has often been on how individual stars, all of different masses, differ from one another. Now, in a paper coming out in the journal Nature Astronomy, a team led by Francesco Ferraro uses our understanding of stars to look at how clusters dynamically evolve. Using the Hubble Space Telescope, they looked at a collection of 5 globular clusters in the Large Magellanic Cloud, a nearby irregular galaxy that is visible to anyone living in the Southern Hemisphere. It was already known that younger clusters are all compact. Overtime, stellar interactions will change the clusters’ shapes, with high mass stars sinking to the centers of clusters while lower mass stars are flung outward, and sometimes simply flung away. Exactly where a system is from the point of formation, to the point when all the massive stars are settled into the system’s core is referred to as a cluster’s dynamical age. In this new research, Ferraro and his team layout ways to define the dynamical age of a system by looking at bright blue stars called blue stragglers, that are formed through stellar collisions and stellar cannibalism. Since these stars are known to be large, are of an unusual color, and are easy to see, their positions throughout a globular cluster can be used to measure a clusters dynamical age. This team finds that the dynamical age of a system isn’t related to the physical age of a system. Some systems more quickly shuffle their massive stars to their core, others take more time to settle. With further observations it may become possible to understand all the factors that can affect the dynamical aging process, including starting mass, size, and starting velocity distribution.
For now, it’s nice to know that just like people, the bodies of clusters age at various rates that don’t always precisely follow the ticking of the clock.
In trying to understand our universe near and far, we often use what is closest at hand to understand what is very far away. From using our Sun as a model for understanding all stars, to using our Earth as a model to understand all planets, this habit can sometimes hold us back from understanding weird objects. As scientists we have to always keep an open mind and rely on creativity to help us find explanations to all the weird and wonderful things we see.
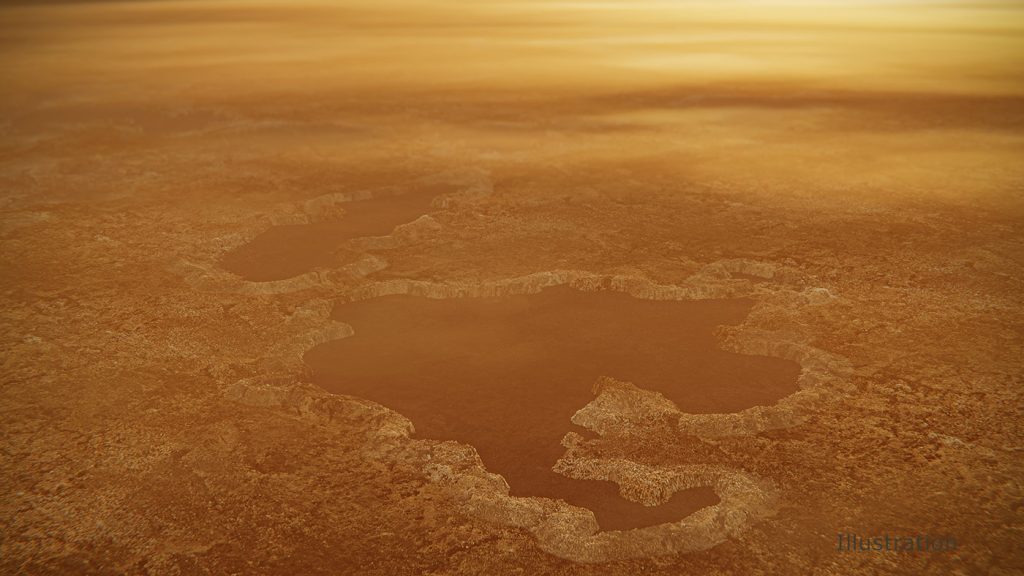
This kind of creativity was needed to understand the surface of Saturn’s moon Titan. The Cassini spacecraft was able to collect data from radar and imaging instruments that describe a world where weather has shaped the landscape, carving out lakes and even river deltas. On Titan, the combination of low gravity and low temperatures allow methane and ethane to fill the role water fills here on Earth. We see clouds, the results of rain or snow fail, and even lakes that liquid methane has carved out of the world’s surface of ice and solid organic materials. Here on Earth, we see this kind of phenomena where water carves out karstic lakes in limestone. With both Earth and Titan’s Karstic Lakes, we see flowing liquid carving hard edged lakes into the surface and it flows across and pools on a dissolvable surface.
For a while, it’s been assumed that all the lakes we see on Titan are formed through this pooling process, but not all lakes on Titan actually have the same surrounding features. Many of the smaller lakes on Titan have extremely steep high edges that rise above the world’s surface. These lakes are tens of miles across, and their steep rims didn’t offer an easy way for liquid to flow in.
A new study published in Nature Geoscience suggests that a radically different process may be responsible for forming these lakes. They suggest that rather than being formed by liquid flowing in, these lakes may be formed by an explosive process that pushed outward, raising the lake rims in much the way we see crater rims get formed. Now, this isn’t the kind of combustion explosion that we’re used to think about when someone says explosion; nothing ignited, nothing burned. Rather, they suggest that liquid nitrogen in Titan’s crust went through a phase change when the moon warmed at some point in the past, and that phase change from liquid to gas would have been accompanied by a radical change in the volume of the nitrogen, which can also be described as an explosion.
Titan is a complex world, that has a methane atmosphere that has probably ebbed and flowed over the eons, allowing the temperature to similarly vary with time. In times with less methane, Titan would have cooled, and nitrogen would have dominated its atmosphere, and rained out to form liquid nitrogen lakes.
While this kind of explosive lake formation isn’t something we see on Earth, we are beginning, for the first time on record, to see massive craters forming in the permafrost in Siberia, where methane and other gases are getting released as the permafrost melts. This is just more reminder that geologic processes follow the same physical laws on every world, large and small, cold or hot, all across our solar system and beyond.
Trackbacks/Pingbacks