Media
Transcript
Today is going to be pretty much a single story day because the story we have is big enough that we need the entire episode to really explain it. This is the story of the discovery of an intermediate black hole with a mass of roughly 142 solar masses that formed via the combination of a 66 and 85 solar mass black hole.
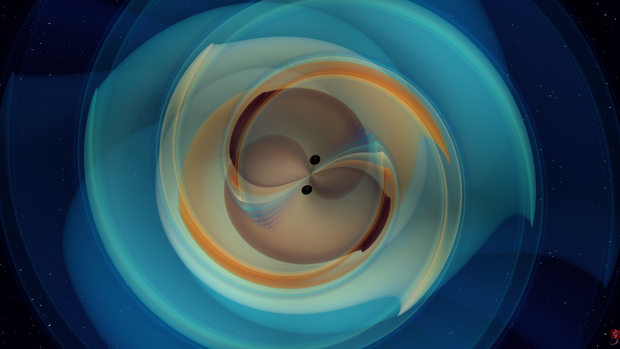
Now, I know some of you are thinking, “We know there are supermassive black holes that are millions and billions of solar masses. Why is a hundred-something solar mass black hole so exciting?
Well, in part, it’s exciting because we haven’t had definitive proof of this size black hole existing.
It appears that black holes can form in at least two different ways. The giant black holes in the largest galaxies that formed at the beginning of the universe appear to have formed through a massive and potentially turbulent inflow of material at the center of the overdensities of material. In this way, the first galaxies and first supermassive black holes all grew in size together.
Once stars began to form, the other confirmed way to form black holes started as well. The most massive stars rapidly fuse successively heavier elements in their cores until their cores are full of iron and energy can no longer be released in fusion processes. These stars, which may start out twenty or more times the mass of the sun, will undergo mass loss throughout their lives, and the final black hole may be as small as 3.5 solar masses, with most stellar-mass black holes being 5-10 solar masses.
The question on many cosmologists’ minds has been: how do we get new galaxies growing and containing growing supermassive black holes in the process? When we look at dwarf galaxies – the kind of galaxies we think are the building blocks of most large galaxies – we aren’t finding black holes in their centers. This implies that, somehow, black holes between stellar-size and supermassive-size have to have a way of forming and growing, allowing new galaxies to form and grow around them.
And these intermediate-mass black holes have refused to be found… until now.
We can detect black holes in two different ways. First, we find them by looking for places in space where only a high-density massive object can explain observed phenomena like accretion disks and high speed orbiting companions. The second discovery method involves looking for the gravitational waves generated when black holes form and merge with other massive objects, including other black holes.
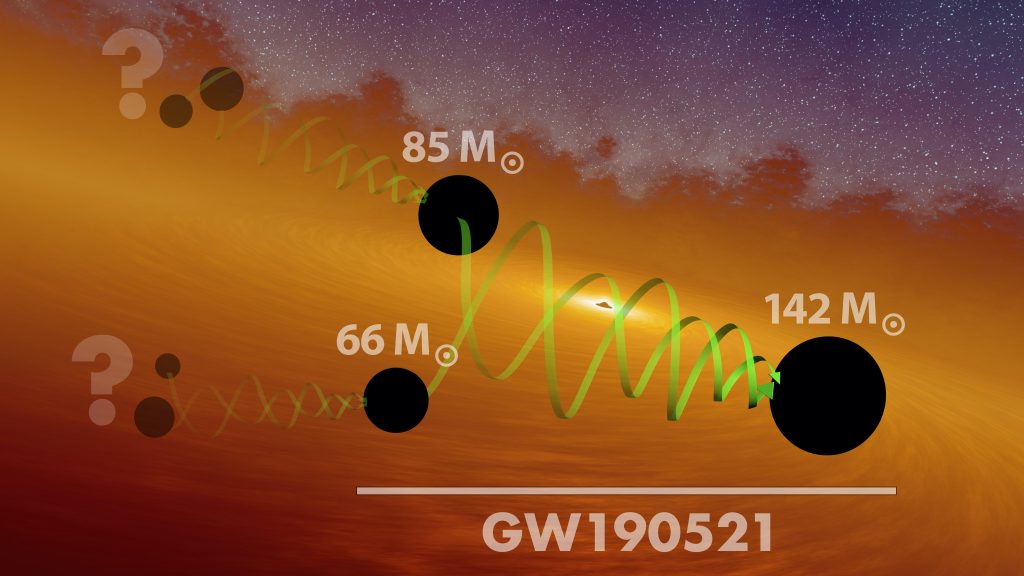
Gravitational waves are literal waves in the space-time continuum that stretch and construct everything as they radiate through space, carrying with them energy lost during the merger or collapse. We detect these waves by looking for the literal change in the distance that occurs between two points in a massive laser system here on Earth. By building multiples of these systems scattered across our planet, scientists can confirm they are detecting real events and use wave arrival times to figure out the approximate direction the wave is coming from. The combined frequency and amplitude pattern of the incoming waves allows scientists to figure out the approximate sizes of the combining objects.
The US detectors are the LIGO systems, and since their first detections in 2015, LIGO, working in collaboration with Europe’s VIRGO system, has made dozens of detections and candidate detections of gravitational waves from merging black holes and neutron stars. The majority of the confirmed detections have come from stellar-mass black hole mergers with systems just tens of solar masses in size. While resulting black hole masses had been as great as 80 solar masses, the initial black holes had all been below the theoretical limit of 65 solar masses, which is thought to be the maximum size black hole a single collapsing star can form.
This new merger – between a 65 and 82 mass black hole – has two inexplicably large black holes.
While a 130 solar mass star can end its life as potentially a 65 solar mass black hole, large stars – stars initially between 120 and 200 solar masses – are unstable and should explode and leave nothing behind. It is unclear if mass loss allows more massive stars to stay massive long enough to form more massive black holes. If this is possible, those stars would create 120 solar mass black holes, leaving a gap of no black holes between 65 and 120 solar masses that form through the collapse of a single star.
For this new merger, these limitations mean that it is just on the edge of the possibility that its 66 solar mass smaller black hole formed from a single star, but that 85 solar mass monster must have resulted from a prior merger. I honestly feel this is most likely a system in which each black hole grew through the merger of two or more lower-mass black holes or neutron stars, giving us the 142 solar mass intermediate black hole we’ve been looking for.
You can read about these results in a pair of papers in The Astrophysical Journal Letters and Physical Review Letters, both authored simply by the LIGO and VIRGO collaborations.
It’s unclear how black holes find one another across the merging generations, and we still have no clear idea of how often this happens. We still have a lot to learn and observe, but now we know these mergers are happening, growing bigger and bigger black holes over time.
Complicated systems excite scientists, and gravitational waves hit all the right buttons: they require amazingly sophisticated instruments, they are understood with truly complicated maths, and they are relativistic systems. But as complicated as gravitational wave systems are, they are still solvable.
What is not solvable is the majority of three-body systems – systems containing three different masses.
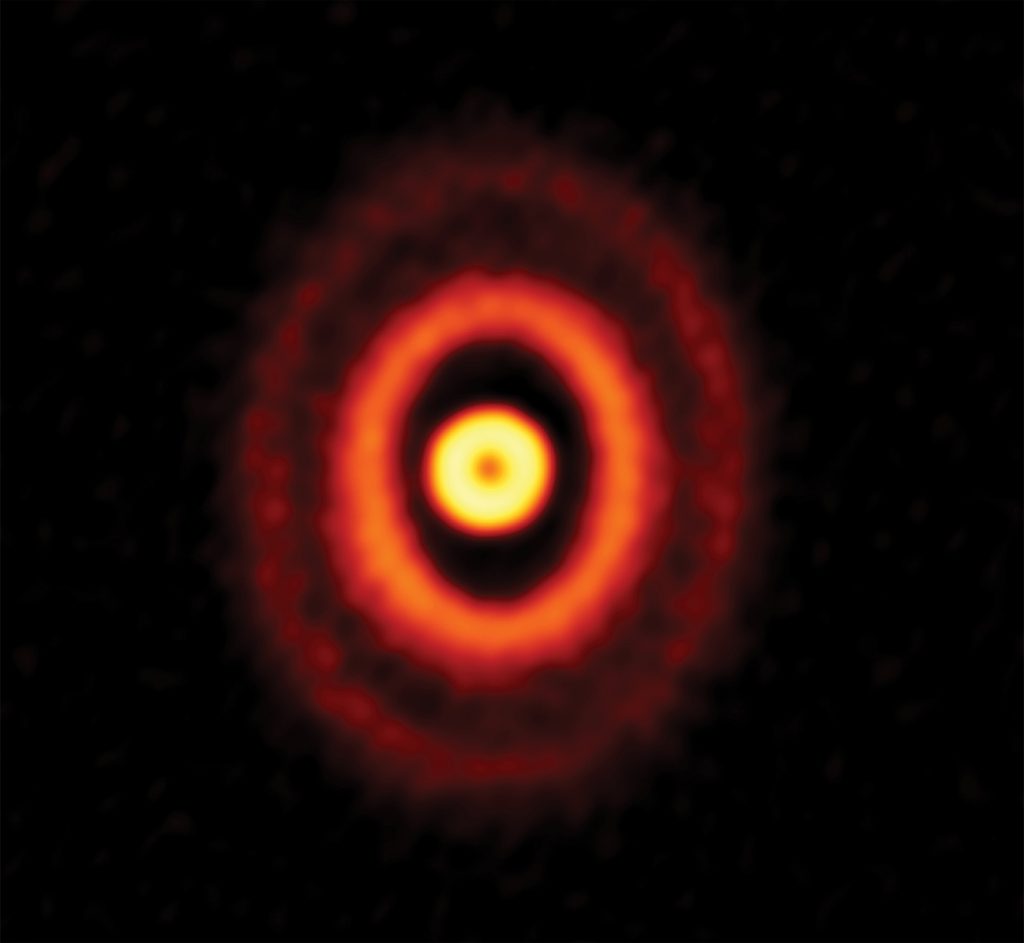
A new triple system has now been discovered by the ALMA Observatory, and this stunning system contains misaligned rings of dust that have the potential to form planets. This system, GW Ori, has three inner stars, with the inner two orbiting 1 AU apart, and the outer star orbiting the inner two from a distance of 8 AU. These are comparatively the distances between the Earth and Sun, and slightly less than the Earth-Saturn distance when they’re closest. Surrounding these stars, ALMA has been able to resolve three misaligned dust rings that can’t be explained by just the stars’ motions. The innermost ring is tilted out of the plane of the outer two rings. All three dust rings contain sufficient material to form planets.
According to team member Nienke van der Marel: Our simulations show that the gravitational pull from the triple stars alone cannot explain the observed large misalignment. We think that the presence of a planet between these rings is needed to explain why the disk was torn apart. This planet has likely carved a dust gap and broken the disk at the location of the current inner and outer rings.
This work appears in The Astrophysical Journal with first author Jiaqing Bi, and it poses more questions than it answers. We can’t wait to see what we learn about this system over time.
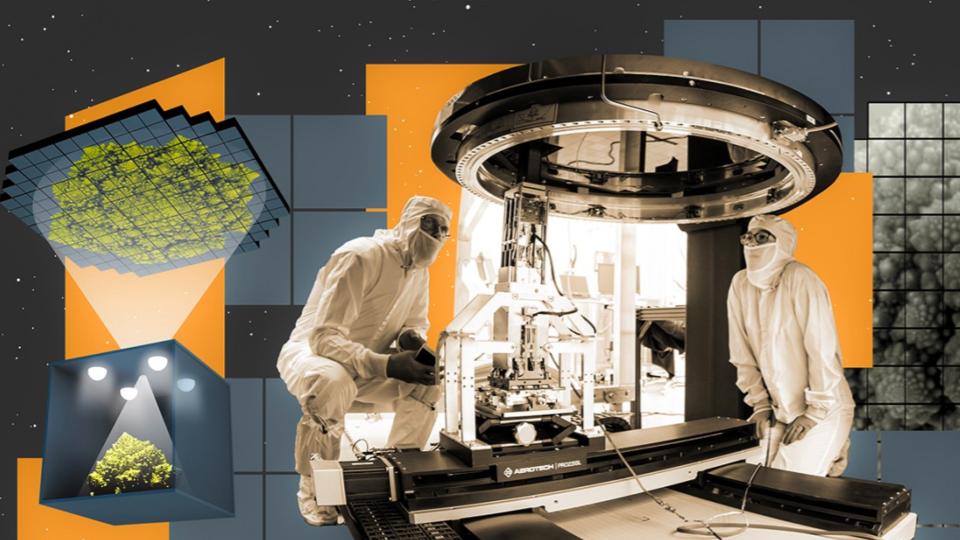
In our final news, the massive camera that will someday be installed on the LSST at the Vera Rubin Observatory has been successfully tested at Stanford University’s Linear Accelerator lab (SLAC). This is the largest camera built to date and will have the capacity of imaging an area of the sky equivalent to forty Moon faces and making out details the size of a golf ball seen from fifteen miles away. The team used a 150-micron wide pinhole camera to direct light reflected off a head of broccoli onto the camera. We’re not entirely sure how they decided on a head of broccoli, and for now, that will remain a mystery for another day.
Learn More
Scientists Detect First Intermediate-Mass Black Hole
- LIGO press release
- Albert Einstein Institute press release
- Northwestern University press release
- The Australian National University press release
- CNRS press release
- “GW190521: A Binary Black Hole Merger with a Total Mass of 150 M_Sun,” LIGO Scientific Collaboration and Virgo Collaboration, 2020 Sep. 2, Physical Review Letters
- “Properties and Astrophysical Implications of the 150 M_Sun Binary Black Hole Merger GW190521,” LIGO Scientific Collaboration and Virgo Collaboration, 2020 Sep. 2, Astrophysical Journal Letters
Misaligned Rings in Planet-Forming Disk Around Triple Stars
- NRAO press release
- ESO press release
- NAOJ press release
- Carnegie Institute for Science press release
- “A Triple Star System with a Misaligned and Warped Circumstellar Disk Shaped by Disk Tearing,” S. Kraus et al., 2020 Sep. 3, Science
- “GW Ori: Interactions Between a Triple-Star System and Its Circumtriple Disk in Action,” J. Bi et al., 2020 May 20, Astrophysical Journal Letters
World’s Largest Digital Camera Snaps First Images
Credits
Written by Pamela Gay
Hosted by Pamela Gay
Audio and Video Editing by Ally Pelphrey
Content Editing by Beth Johnson
Intro and Outro music by Kevin MacLeod, https://incompetech.com/music/