Media
Transcript
It seems we have to start nearly every episode with the caveat that this is 2020, and the timeline is, well, not good. Let me reassure you all that Dr. Pamela is fine but a bit under the weather, and we’re taking this opportunity to throw me at the wall, so to speak, and see if I stick. She’s here producing, and I’m here feeling nervous and excited. If this goes even the smallest bit okay, you may see more of me.
Speaking of the weather, while planet Earth has been dealing with Hurricane Isaias, we turn instead to another planet full of storms – Jupiter.
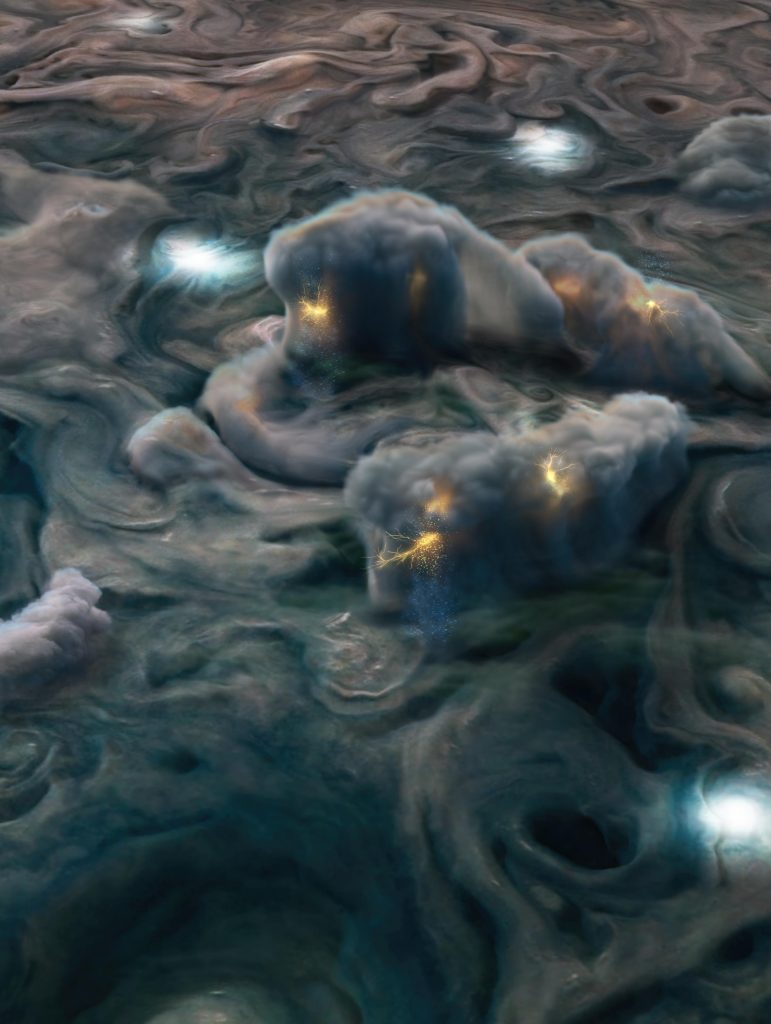
In a trio of papers released yesterday in Nature and the Journal of Geophysical Research: Planets, researchers used data from NASA Juno’s microwave radiometer and found evidence of ammonia-rich hail and shallow lightning.
On Earth, lightning is thought to occur where water exists in all three elemental states – solid, liquid, and gas. Storms on Jupiter work in a similar fashion, especially deep in the atmosphere where the temperature can be close to the freezing point of water, except that here, the storms circulate water-ice crystals up into the higher atmosphere where it meets a layer of ammonia vapor, which acts as an antifreeze.
The ice crystals then partially melt, and the water mixes with the ammonia. These new crystals get heavier and begin to sink back into the cloud layer, acquiring a thicker and thicker layer of water ice. The deeper these “mushballs” fall into Jupiter’s atmosphere, the warmer they get, and the ice crust melts until both the ammonia and the water ice evaporate into the lower atmosphere.
Which brings us to how the ammonia mixing is important. Scientists have been pondering why the amount of ammonia in the atmosphere varies across Jupiter. It turns out that these mushballs are depleting the deep atmosphere of its ammonia as storms circulate the crystals up and down. So where there are thunderstorms, the ammonia levels drop over time, causing the variability we have seen in previous data.
But what about the lightning?
Juno’s cameras observed small flashes of lightning in the cloud tops, and because Juno is closer to the planet than previous missions, it can detect even smaller and shallower flashes than before. Since these newly observed flashes are occurring in regions where water alone is not found in its liquid state, and since we have already explained that lightning needs all three states to occur, the researchers determined that the liquid ammonia-water mix may be the source of that third elemental state.
Any new understanding of how a planet works is amazing to me, and we can someday apply these lessons to exoplanets.
For right now, we’ll have to stick closer to home and keep learning more about our own solar system, which is why we have spacecraft like Juno, that get up close and personal with the planets they explore, allowing us to study features we can’t see from our perspective on Earth.
Worlds without robot explorers are harder to understand. For most of the modern era of space exploration, Venus has been left alone but that changed in 2015 when the Japanese Venus Climate Orbiter, or Akatsuki, inserted itself into orbit. The results of the past five years of data are starting to pay off in paper after paper, and today in the Geophysical Research Letters, a new paper by Javier Peralta and company announces the discovery of undulating structures in Venus’ clouds that last for decades at a time.
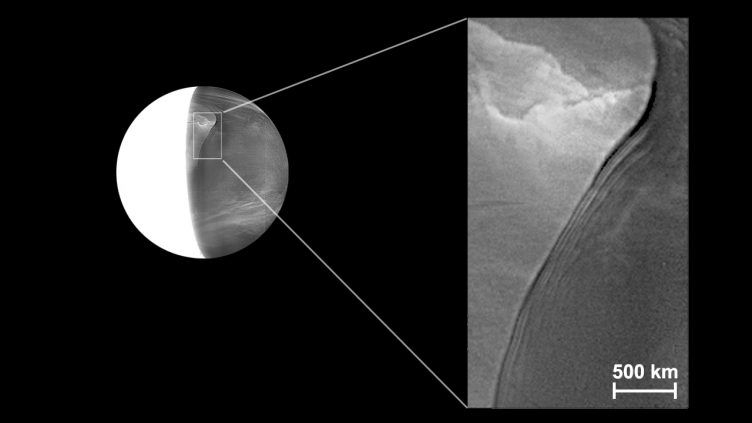
According to Peralta: Since the disruption cannot be observed in the ultraviolet images sensing the top of the clouds at about 70 kilometers height, confirming its wave nature is of critical importance. We would have finally found a wave transporting momentum and energy from the deep atmosphere and dissipating before arriving at the top of the clouds. It would, therefore, be depositing momentum precisely at the level where we observe the fastest winds of the so-called atmospheric super-rotation of Venus, whose mechanisms have been a long-time mystery.
On Earth, the closest we have to this kind of structure is a weather front with a sharp leading edge of clouds. While weather fronts on Earth might span many degrees of latitude, the structure on Venus spans hemispheres, stretching from 30 degrees north to 40 degrees south. Nothing of this proportion has been seen elsewhere in our solar system.
There is much we still don’t know about Venus, and the more papers like this come out, the more it becomes clear that we don’t even know what questions we need to be asking! Here is to hoping we get more Venus missions in the not-too-distant future.
Finally, we are happy to share that another robotic explorer, NASA’s Mars InSight, is making headway on one of its key mission goals: mapping the internal structure of Mars. This lander came equipped with a very sensitive seismograph, capable of actually measuring not just the primary shake of a marsquake, but also the echoes as the seismic waves move multiple times through the planet. This extreme sensitivity allows this single seismograph to determine the sources of quakes and use the timing differences in how different kinds of waves travel through the world to map out density variations within Mars.
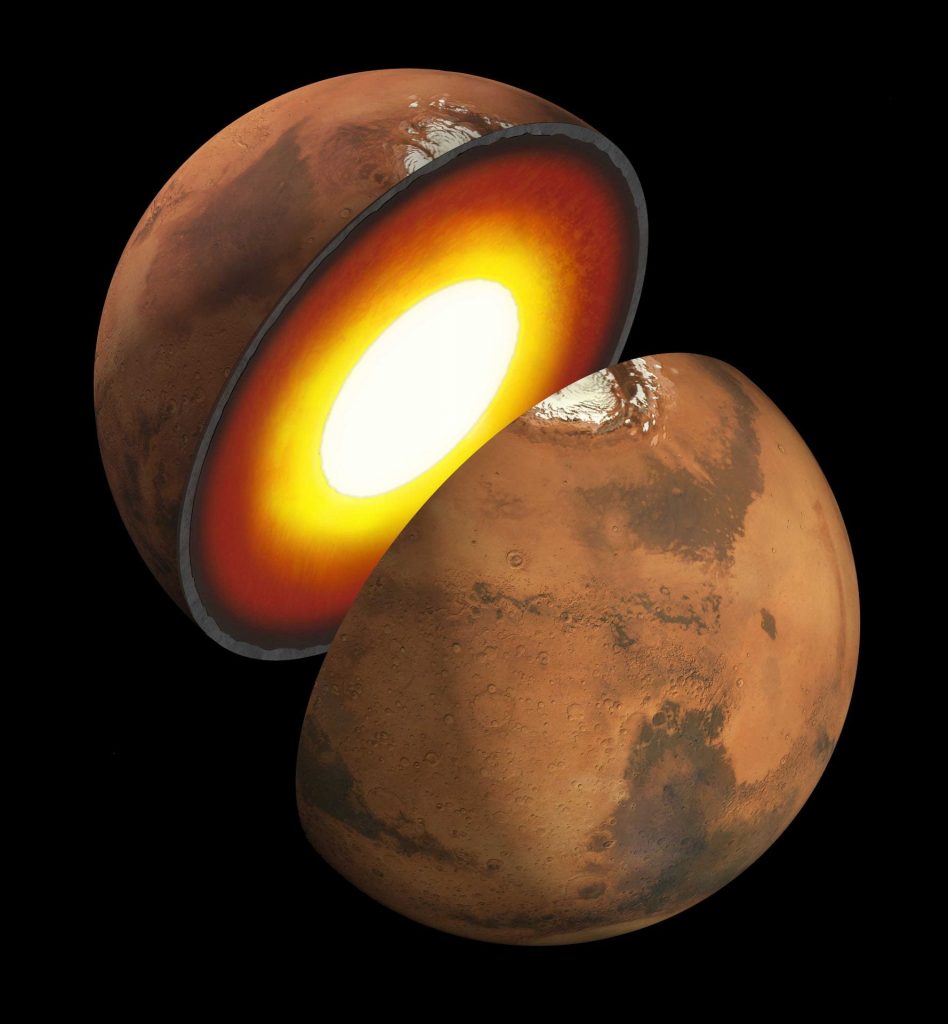
New results, also published in Geophysical Research Letters, by Sizhuang Deng & Alan Levander, find that Mars has three subsurface boundaries – places where the density of the planet shifts. There is a solid inner core, surrounded by a mantle with two density regions, with a crust floating on top. These three measured layers are consistent with prior models that looked at Mars’ moment of inertia and other factors, and allow scientists to directly measure what previously could only be inferred. They found the mantle is roughly 22 miles (35 kilometers) thick at the lander’s location.
The next transition is harder to explain, and here we quote from the Rice University press release: The second is a transition zone within the mantle where magnesium iron silicates undergo a geochemical change. Above the zone, the elements form a mineral called olivine, and beneath it, heat and pressure compress them into a new mineral called wadsleyite. Known as the olivine-wadsleyite transition, this zone was found 690-727 miles (1,110-1,170 kilometers) beneath InSight.
Finally, the boundary between the mantle and Mars’ iron-rich core appears 945-994 miles (1,520-1,600 kilometers) below the lander.
Mars is a large solid ball of rock – complex layered rock – and thanks to InSight, those complexities have been revealed. Way to go, little robot.
Learn More
Ammonia-Rich Hail Sheds New Light on Jupiter’s Weather
- CNRS press release
- NASA JPL press release
- “Storms and the Depletion of Ammonia in Jupiter: I. Microphysics of ‘Mushballs,’” T. Guillot et al., 2020 Aug. 6, Journal of Geophysical Research: Planets
- “Storms and the Depletion of Ammonia in Jupiter: II. Explaining the Juno Observations,” T. Guillot et al., 2020 Aug. 6, JGR Planets
- “Small Lightning Flashes Indicating Shallow Electrical Storms,” H. N. Becker et al., 2020 Aug. 6, Nature
Decades-Long Deep Giant Cloud Disruption Discovered on Venus
- Instituto de Astrofísica e Ciências do Espaço (IA) press release
- “A Long‐Lived Sharp Disruption on the Lower Clouds of Venus,” J. Peralta et al., 2020 May 27, Geophysical Research Letters
NASA’s InSight Lander Used for Deep Mars Measurements
- Rice University press release
- “Autocorrelation Reflectivity of Mars,” Sizhuang Deng & Alan Levander, 2020 Aug. 4, Geophysical Research Letters
Credits
Written by Beth Johnson and Pamela Gay
Hosted by Beth Johnson
Audio and Video Editing by Ally Pelphrey
Content Editing by Beth Johnson
Executive Producer Pamela Gay
Intro and Outro music by Kevin MacLeod, https://incompetech.com/music/