Media
Transcript
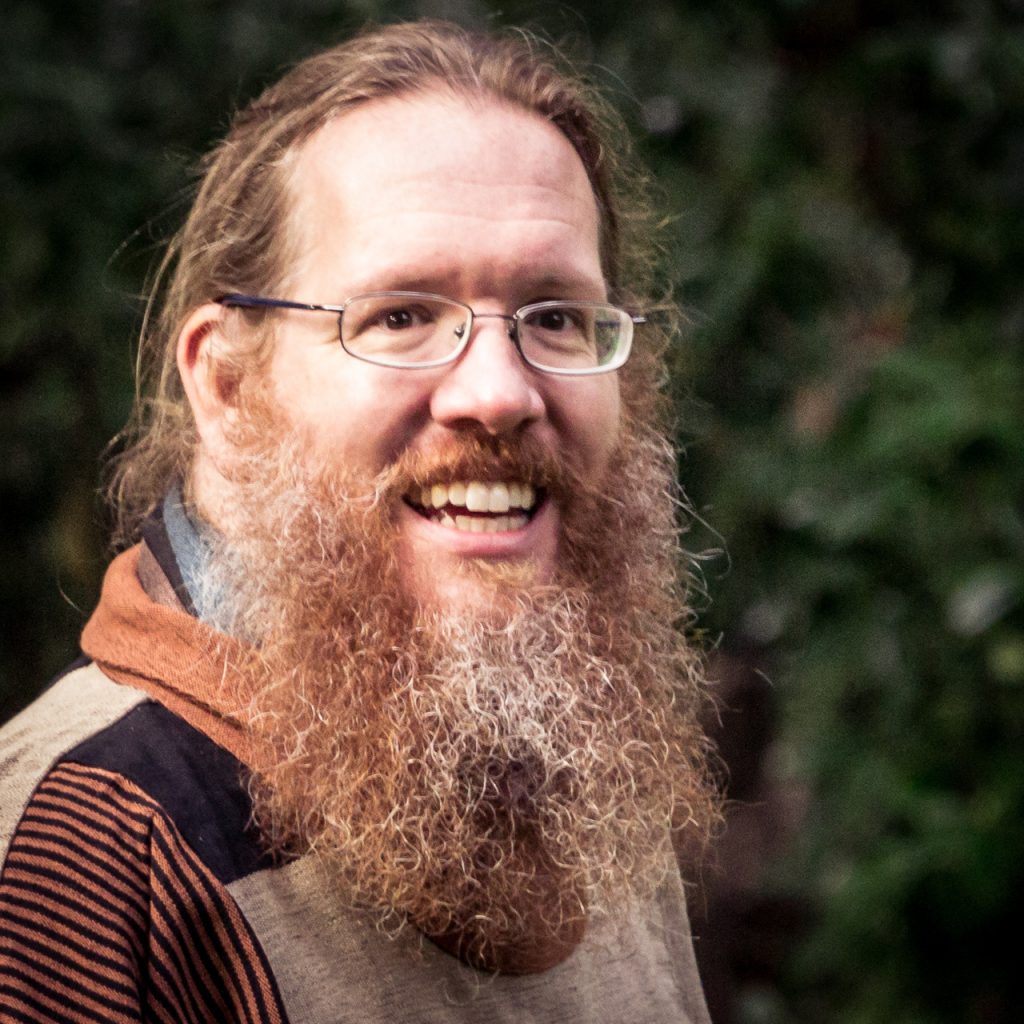
Today we are going to be joined by guest Dr. Matthew Graham. He is a Caltech researcher and the first author on a paper describing the discovery of a transient light phenomenon associated with a black hole merger. This light lagged after the merger by several days and nicely matched earlier predictions of how black hole mergers can interact with their surroundings to shock things into glowing. Anytime a theory is found to be real is an exciting day, and we’re super pleased that Matthew will be able to join us to discuss these results.
Before we get to the interview, however, let’s take a look at today’s news.
In today’s top story, planetary scientists at the University of Warwick have discovered a super weird object that is best explained as a planetary core from a former gas giant.
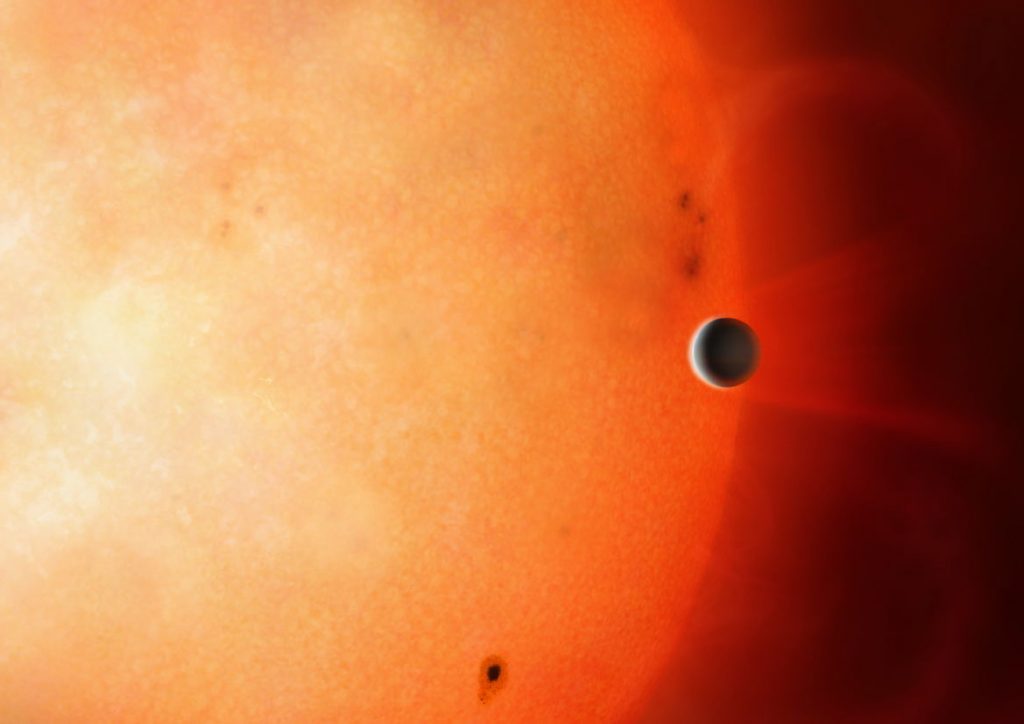
Located 730 light-years away, this object, TOI 849 b, orbits a star not too different from our Sun. The catch is that its atmosphere was completely stripped off, leaving behind an almost forty-Earth-mass core of rock-density material. Today, this world is snuggled so close to its star that it orbits every eighteen hours from a point where it feels the Sun’s every flare. According to the paper’s abstract: The planet could have been a gas giant before undergoing extreme mass loss via thermal self-disruption or giant planet collisions, or it could have avoided substantial gas accretion, perhaps through gap opening or late formation.
You can read the entire paper, with first author David Armstrong, in the journal Nature.
To break that explanation down, they see three different scenarios as possibly producing this atmosphere-less rock: 1) maybe it formed this way, and due to a lack of gas where it formed, it couldn’t build up an atmosphere; 2) maybe this world had a horrible collision with other gas giants and lost its atmosphere during the catastrophe. Those are both fine examples, but it’s the third one that is most tantalizing: 3) this world could be the naked core of a gas giant that had its atmosphere blasted away from its star.
This is a large object, and removing its atmosphere is non-trivial, but with such a small orbit it’s possible. This paper is a reminder that when we look at worlds today, we can’t really know how they started. In devising solar system evolution models, we need to remember that worlds can start with amazing atmospheres that stars and collisions and possibly other events like gamma-ray bursts can all strip away, and collisions with asteroids and comets can bring an atmosphere back. The one constant of the universe is that everything is changing, and what we see today is a snapshot of the changing cosmos.
The dynamic nature of our universe appears in different ways in different objects. If you look at a massive galaxy cluster in optical light, you can often find evidence of trauma: gas and dust torn from their systems and galaxies deformed through close encounters with their neighbors. The true drama, however, pops out in radio light, where the trails of particles and tangled magnetic fields appear to shine.
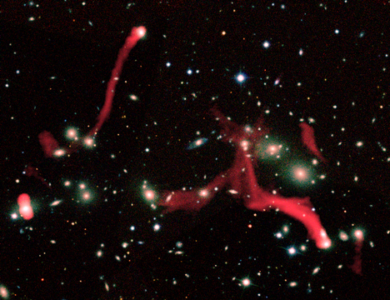
In a new data set on the cluster Abell 2255, a team led by Andrea Botteon captures all these effects in stunning detail using the LOFAR radio telescope. According to team member Gianfranco Brunetti: The filaments discovered by LOFAR could form exactly as a consequence of these turbulent motions. Another possibility that we are considering is that the filaments originate from the interaction between the galaxies, which move at speeds of many hundreds of km/s inside the cluster and the plasma that produces the radio emission of the halo.
The degree of emissions from shockwaves is something that had been somewhat anticipated, but this is the first study to look at how far the radio emissions extend from this cluster. According to team member Reinout van Weeren: One of the goals is to understand if the radio emission extends also beyond Abell 2255, tracing the gigantic cosmic web that connects clusters of galaxies in the Universe.
When it comes to understanding our universe, everything we study needs to be considered in context. This means that when we’re trying to understand the emissions from various sources, we also have to account for other sources changing those emissions along the way. This recently became particularly important for researchers trying to understand how it is that the IceCube Neutrino Observatory keeps observing an unexpectedly large flux of high energy neutrinos with none of the corresponding gamma rays that we expect to see associated with them.
According to team lead Kohta Murase, high-energy cosmic neutrinos are created by energetic cosmic-ray accelerators in the universe, which may be extreme astrophysical objects such as black holes and neutron stars. They must be accompanied by gamma rays or electromagnetic waves at lower energies and sometimes even gravitational waves. So we expect the levels of these various ‘cosmic messengers’ that we observe to be related.
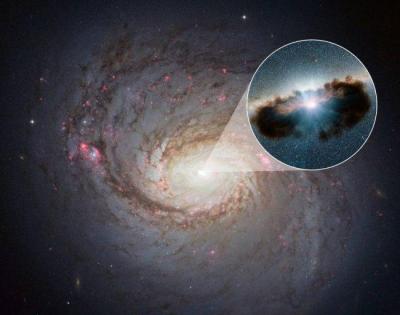
Interestingly, the IceCube data have indicated an excess emission of neutrinos with energies below 100 teraelectronvolts (TeV), compared to the level of corresponding high-energy gamma rays seen by the Fermi Gamma-ray Space Telescope. This kind of “what the?” moment implies we either don’t fully understand the origins of high-energy neutrinos, or there is something else involved.
Neutrinos readily pass through materials, while gamma rays can be absorbed by gas, dust, and other intervening material. So what is eating the gamma rays? In a new paper in Physical Review Letters, this research team describes how the environment around supermassive black holes can both generate the high-energy neutrinos and gamma rays and then absorb the gamma rays into the surrounding accretion disk. Active galactic nuclei – feeding central black holes in galaxies – can both produce the needed particles and have the needed absorbing material, neatly explaining a whole lot of previously confusing data.
Science tends to work in two directions: we both observe things and then try to explain what we observe, like with the IceCube results, and we also make predictions about the universe and look for evidence of those predictions. Some theories are harder to believe than others, even with a profundity of evidence, and these are the ones I get the most excited to see proved true once again. From relativity getting tested over to quantum mechanics getting tested over and over, it is just pleasing when reality says “I know I’m weird, but I’m predictably weird.” The LIGO experiments have just allowed one of these amazing tests of quantum mechanics to take place.
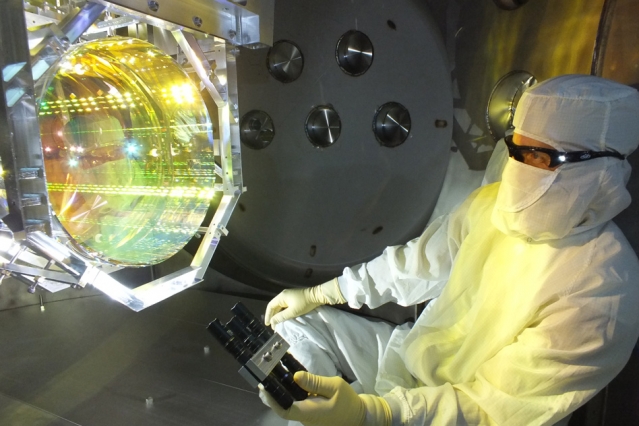
It had been predicted that objects the size of LIGO’s 40-kg mirrors would jiggle at the smallest level due to the constant popping in and out of existence of virtual particles. The amount that it moves is a mind-blowingly small amount: just 10^-20 of a meter. Researcher Lee McCuller uses the following analogy: A hydrogen atom is 10^-10 meters, so this displacement of the mirrors is to a hydrogen atom what a hydrogen atom is to us. Thanks to the extraordinary preciseness needed to measure gravitational waves, this amount of noise is apparent in the LIGO data, and nicely matches predictions, showing once again that with science we can understand things that our day-to-day experience never prepared us for.
And this circles nicely back to LIGO, and our guest for the day, Dr Matthew Graham. In just a few moments we’ll be discussing how a black hole merger can kick things into shining.
Learn More
Exposed Planetary Core Allows Glimpse Inside Other Worlds
- The University of Warwick press release
- “A Remnant Planetary Core in the Hot-Neptune Desert,” David Armstrong et al., 2020 July 1, Nature
The Beautiful Mess in Galaxy Cluster Abell 2255
- European Astronomical Society press release
- “The Beautiful Mess in Abell 2255,” A. Botteon et al., 2020, to appear in the Astrophysical Journal (Preprint on arxiv.org)
Excess Neutrinos and Missing Gamma Rays? Blame Black Holes!
- Penn State Eberly College of Science press release
- “Hidden Cores of Active Galactic Nuclei as the Origin of Medium-Energy Neutrinos: Critical Tests with the MeV Gamma-Ray Connection,” Kohta Murase, Shigeo S. Kimura & Peter Mészáros, 2020 June 30, Physical Review Letters (Preprint on arxiv.org)
Quantum Fluctuations Can Jiggle Human-Scale Objects
- MIT News release
- “Quantum correlations between light and the kilogram-mass mirrors of LIGO,” Haocun Yu et al., 2020 July 1, Nature
Credits
Written by Pamela Gay
Hosted by Pamela Gay
Audio and Video Editing by Ally Pelphrey
Content Editing by Beth Johnson
Intro and Outro music by Kevin MacLeod, https://incompetech.com/music/