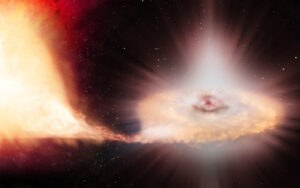
As a scientist, I get a lot of weird hate mail. There are the folks telling me relativity is wrong. There are the folks telling me NASA is keeping secret something like the Earth is flat or the Senate has been taken over by aliens. And then there are the folks telling me that I need to open my eyes and look at the data because scientists are otherwise too closed-minded to notice what our data is telling us.
Every time I get the digital “why can’t you scientists just look at the data” lecture, it makes me wonder what people think scientists do because all we do is look at data, and when that data tells us our understanding of the universe is wrong, we’re pretty good at accepting the data and throwing out our false understandings… even when the data makes our life a whole lot harder.
Case in point: back in 1998, two different supernovae research teams both found in their data evidence that our universe is expanding at an accelerating rate. The prior theory had indicated that the universe would forever expand at something close to a constant rate, slow to essentially a stop, or eventually reverse course and collapse in on itself. The idea that it could expand at an accelerating rate was just a footnote – sure the math allows this but this isn’t math you need to explore because it just ain’t so – It was something so ridiculous as to just be ignorable.
Which I was fine with — the maths of an accelerating expanding universe are not something I wanted to ever solve and had we thought that option was possible, someone would have made solving it a homework problem.
But with those two data sets published in 1998, we had to redo everything. Just thirteen years later – an eye blink in the career of a researcher – the phenomena was generally accepted, blamed on a mysterious something we called dark energy, and the Nobel Prizes were handed out.
Scientists are damn good at looking at our data and recognizing when we need a new theory.
And we still need a new theory.
Since that cosmology-disrupting discovery, the field of astronomy has made understanding the nature of dark energy a priority, and this has included looking for new ways to measure cosmic expansion, verifying the assumptions made in past data sets, and in otherwise trying to figure out what new data we need to acquire.
One of the major projects in this search for dark energy has been the Dark Energy Survey. Using what were the most advanced cameras of their time, an international collaboration surveyed 5,000 square degrees of the southern sky to look for supernovae and to map out the large-scale structure of the universe.
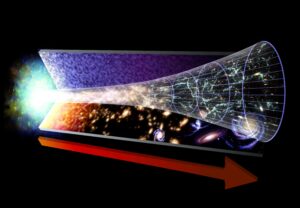
After the Big Bang, our universe was an almost smooth distribution of energy. Over time, that distribution got lumpier, and a lot of the energy solidified out into matter which then got lumpier and lumpier as it formed into clouds, then stars and galaxies, and then clusters of galaxies that formed even larger walls and superclusters of galaxy clusters. As we look at volumes of space that are further and further away, we get to essentially look back in time.
The further away we look, the longer it takes the light to reach us, and we get to experience the past in our far-off glances. We see the Sun as it was eight minutes ago; Pluto’s light is from hours ago. The stars are years and centuries of light travel time away.
Telescopes are time machines that only ever look backward.
With their amazing 570-megapixel camera, the 400 scientists from 25 institutions that make up the DES Collaboration have been able to look back in time and see large-scale structures grow. So far, this part of the survey hasn’t radically changed our understanding, but it provides us a baseline understanding of what our theories must explain if they are valid.
And this is one heck of a super important start.
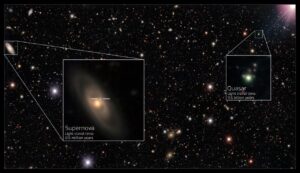
Along with their mapping, the Dark Energy Survey also looked at key areas of the sky once a week to explore for supernovae to see if they could further refine the work done by those to Nobel Prize-winning teams in 1998.
In a new paper in The Astrophysical Journal (pre-print), the Dark Energy Survey team has published their final catalog of supernova results. This release contains 1,500 new high-redshift type Ia supernovae — the kind formed when white dwarf stars are fed too much and explode. By measuring both the apparent distance to each explosion based on how bright they appear and the recession velocity of each point of light, we can plot the expansion rate as a function of distance. There is a lot of error in these measurements — the supernovae have orbital velocities within their galaxies and their galaxies have velocities within their clusters. Some systems are just a bit brighter or fainter naturally. Some are just weird stars that defy proper behavior.
Stars are messy.
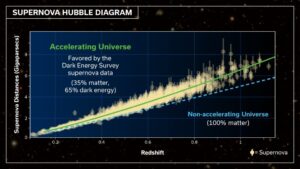
But within the statistical noise of all these explosions is one simple truth: The data is completely consistent with prior observations. Our universe appears to be expanding at a rate that increases, inexplicably, over time.
The weirdest thing about this is the dark energy seems to be constant with volume, such that as the universe expands, the dark energy stays the same density everywhere all the time, without getting diluted. This is like imagining an expanding room with no way in or out that stays knee-deep in water no matter how big the room gets. Sure, if there was a vent for water to get added to the room constantly, this might make sense. But in a closed room, how does the depth of water stay constant as the room expands?
We don’t understand dark energy at the level of not knowing if it is just some intrinsic thing that space just has, if it is a force, a pressure, or a leak of energy from the other dimensions we don’t generally experience. We just don’t know.
But the DES has shown us it really is there and data set after data set are showing us pretty much the same thing. Hopefully, either time or additional datasets will help theorists find that bit of inspiration they need to figure out what factor is driving our universe apart.
Caught in the Act: Neutron star formation
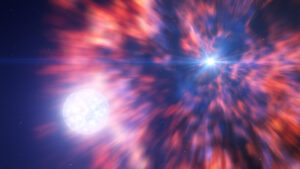
The supernovae used to study the expansion of our universe – type Ia supernovae – are a case of small objects exploding when overfed. These explosions don’t leave anything particularly chunky behind. Sure, there will be a supernova remnant and likely bits and bobs of former solar systems, but when that white dwarf goes boom, the white dwarf goes away. But this is just one kind of supernova.
Most kinds of supernovae are formed when a massive star runs out of fuel to use to produce light in its cores. During nuclear fusion, lighter elements are smushed together into progressively more massive atoms. For things like hydrogen, carbon, and other small atoms, this process releases energy; essentially, it takes less energy to have one He-3 atom than it does to have three different hydrogen atoms, and that difference in energy is released as light. The light then creates a pressure that supports the star against gravity trying to collapse the star.
For the most massive stars, fusion can keep going until iron. It turns out that atoms heavier than iron require energy to be added to fuse them, so at iron, fusion stops being useful, and a core that doesn’t have fusion going on is going to stop generating light, stop supporting the outer layers of the star against gravity, and it’s going to collapse, which is a process that admittedly adds energy into the system — so much energy that everything explodes as a supernova.
According to theory, some of these stars will form neutron stars, some will form black holes, and some will simply blast themselves apart entirely. Since we see supernova remnants containing neutron stars, we’ve put a lot of faith in these theories, but we haven’t been able to see a system go from star to supernova remnant with a neutron star… at least not until now.
In a pair of new papers published in Nature and The Astrophysical Journal Letters, researchers led by Thomas Moore and Ping Chen show that SN 2022jli appears to have left behind a compact object that is a neutron star — a hungry neutron star that is making itself known by taking chunks out of its companion.
As the story goes, one star in a two-star binary system exploded in May 2022. This particular system was in the nearby galaxy NGC 157, which makes it easy to observe. During the supernova, the smaller, less explody star took a blow that caused its outer atmosphere to puff out. The compact object left behind when the larger star exploded then began to orbit through this puffed-out atmosphere, stealing material with its gravity and pulling a hot disc of material about itself. While the compact object is so far hidden, only a neutron star or possibly a small black hole can explain everything they are currently seeing.
Short of having a supernova go off in our galaxy close enough to resolve a left-behind neutron star, this is as close as we can expect to get to seeing a neutron star form.
Sometimes the universe and the theorists are in perfect alignment.